How Quantum Computers Break The Internet... Starting Now
TLDRThis video explores the emerging threat of quantum computing to current encryption methods, detailing the Store Now, Decrypt Later (SNDL) strategy by adversaries. It highlights the evolution of encryption from symmetric to asymmetric systems like RSA, which relies on the difficulty of factoring large prime numbersโa task quantum computers could drastically simplify. The script discusses how quantum computers use superposition and entanglement to perform calculations in parallel, potentially breaking RSA encryption. It concludes by introducing efforts to develop quantum-resistant encryption algorithms, with NIST selecting four algorithms based on lattice mathematics, illustrating the ongoing battle to secure data against future quantum capabilities.
Takeaways
- ๐ป Nation states and individual actors are storing encrypted data today to decrypt later with quantum computers, a strategy known as Store Now, Decrypt Later (SNDL).
- ๐ Quantum computers pose a threat to current encryption methods by potentially breaking them within decades, leading to legislative actions for cryptographic transition.
- ๐ค Symmetric key algorithms require sharing a secret key in person for secure communication, which is impractical for distant parties.
- ๐พ RSA encryption, invented by Rivest, Shamir, and Adleman, uses two large prime numbers to create public and private keys, revolutionizing digital security without the need for shared secret keys.
- ๐ฅ Quantum computers operate using qubits that can exist in multiple states at once, enabling them to perform many calculations simultaneously and potentially break current encryption.
- ๐ Peter Shor's algorithm on a quantum computer can factor large numbers quickly, undermining the security of RSA and other public key cryptosystems.
- ๐จ Lattice-based cryptography is emerging as a quantum-resistant encryption method, using high-dimensional lattices to create problems challenging for both classical and quantum computers.
- ๐ National Institute of Standards and Technology (NIST) is leading a global effort to standardize post-quantum cryptographic algorithms, selecting candidates likely to withstand quantum attacks.
- ๐ The transition to quantum-resistant encryption is critical for protecting sensitive information against future quantum computing capabilities.
- ๐ฑ Educating oneself about quantum computing and encryption is important as these technologies will significantly impact future digital security.
Q & A
What is the 'Store Now, Decrypt Later' (SNDL) strategy and why is it used?
-SNDL is a strategy where nation states and individual actors collect and store encrypted data, such as passwords and bank details, despite currently being unable to decrypt it. They do this because they anticipate that within the next 10 to 20 years, quantum computing advancements will enable them to decrypt this information quickly, making even today's secure data vulnerable in the future.
How does quantum computing pose a threat to current encryption methods?
-Quantum computing poses a threat because it operates on qubits that can exist in multiple states simultaneously, allowing for the execution of many calculations at once. This capability can potentially break the encryption of data secured by today's algorithms, which are not designed to withstand the parallel processing power of quantum computers.
What legislative action has the US Congress taken in response to the quantum computing threat?
-In response to the threat posed by quantum computing, the US Congress has passed legislation mandating all agencies to start transitioning to new methods of cryptography that cannot be broken by quantum computers. This proactive measure aims to secure data against future quantum attacks.
What makes RSA encryption vulnerable to quantum computing?
-RSA encryption is vulnerable to quantum computing because it relies on the difficulty of factoring large prime numbers, a task that classical computers find extremely time-consuming. Quantum computers, however, can efficiently solve this problem using algorithms like Shor's algorithm, which can factor these large numbers much more quickly.
How do quantum computers perform calculations differently from classical computers?
-Quantum computers perform calculations using qubits, which can represent both 0 and 1 simultaneously through superposition. This allows them to process multiple possibilities at once, unlike classical computers which process one state at a time. Quantum algorithms leverage this capability to perform complex calculations more efficiently.
What is the significance of the quantum Fourier transform in quantum computing?
-The quantum Fourier transform is significant because it enables the extraction of frequency information from a periodic superposition, a key step in solving problems central to cryptography. This ability is crucial for algorithms like Shor's, which relies on it to factor large numbers efficiently.
What are some challenges in utilizing quantum computers for practical applications?
-One major challenge is that while quantum computers can generate a superposition of all possible outcomes, measuring this superposition collapses it to a single outcome, losing all other information. Therefore, a smart way to convert a superposition into a useful form without losing information is needed, which is a non-trivial task.
What progress has been made towards quantum-safe encryption algorithms?
-In 2022, the National Institute of Standards and Technology (NIST) selected four algorithms as part of their post-quantum cryptographic standard, focusing on encryption methods that are secure against both classical and quantum computing threats. These algorithms are primarily based on the mathematics of lattices.
How do lattice-based encryption algorithms work?
-Lattice-based encryption algorithms rely on the complexity of finding the shortest path in a high-dimensional lattice. While it is easy to create a lattice and difficult paths within it, solving for the shortest path without specific knowledge (the secret key) is computationally infeasible, even for quantum computers.
Why is the transition to quantum-safe encryption important?
-The transition to quantum-safe encryption is crucial to protect against future quantum computing threats. As quantum computers become more capable, they will be able to break current encryption standards, potentially exposing sensitive data. Transitioning to quantum-safe encryption ensures long-term data security.
Outlines
๐ The Quantum Threat to Encryption
This section explains the 'Store Now, Decrypt Later' (SNDL) strategy, where nation states and individuals collect encrypted data with the expectation that future quantum computers will crack current encryption methods. It highlights the significance of encryption in protecting sensitive information like bank details and government intelligence, and acknowledges the efforts and concerns of governmental bodies like the National Security Agency regarding the vulnerability of public key algorithms to quantum computing. It ends by noting the U.S. Congress's response in mandating a transition to quantum-resistant cryptographic methods.
๐ Quantum Computing Basics
This part delves into the principles of quantum computing, contrasting it with classical computing by explaining bits vs. qubits and the concept of superposition. It outlines the potential of quantum computers to perform multiple calculations simultaneously, vastly outperforming classical computers for certain tasks. The emphasis is on how quantum computing could impact cryptography, particularly the RSA encryption method, by using superpositions to factor large numbersโsomething infeasible with current classical computing.
๐ก Quantum Cryptanalysis
Here, the focus shifts to how a quantum computer could factorize large numbers, exploiting the properties of quantum mechanics. It describes a simplified example to explain the process, including selecting a number, finding its power cycle, and then using Euclid's algorithm to determine the prime factors of a given number. This explanation lays the groundwork for understanding the quantum factorization method, demonstrating its efficiency compared to classical approaches.
๐งฉ Quantum Computing and Encryption
This section illustrates the practical application of quantum computing in breaking encryption through an example involving modular arithmetic and the use of qubits in a quantum computer. It explains the quantum Fourier transform's role in identifying the periodicity of remainders, thereby aiding in the factorization of large numbers. The narrative conveys the complexity of executing this process and the substantial number of qubits required, touching on the advancements and challenges in quantum computing technology.
๐ Towards Quantum-Resistant Encryption
This concluding part discusses the search for encryption methods immune to quantum computing's potential threats. It introduces the National Institute of Standards and Technology's (NIST) competition to develop post-quantum cryptographic standards and highlights the selection of algorithms based on lattice mathematics as promising solutions. The example provided explains how these algorithms function and their inherent resistance to both classical and quantum decryption methods. It underscores the ongoing effort by researchers to safeguard data against future quantum attacks.
Mindmap
Keywords
๐กQuantum Computing
๐กEncryption
๐กStore Now, Decrypt Later (SNDL)
๐กRSA Encryption
๐กQuantum Bits (Qubits)
๐กPublic Key Cryptography
๐กShor's Algorithm
๐กPost-Quantum Cryptography
๐กLattices in Cryptography
๐กSymmetric Key Algorithm
Highlights
Nation states and individual actors are storing encrypted data, believing future quantum computers will decrypt it.
Store Now, Decrypt Later (SNDL) strategy anticipates quantum computing advancements within 10-20 years.
US Congress mandates transition to quantum-resistant cryptography due to SNDL threat.
Introduction of symmetric and asymmetric key algorithms revolutionized digital encryption.
RSA encryption, based on prime numbers, currently underpins public key cryptography.
Quantum computers leverage qubits for parallel processing, challenging current encryption methods.
Peter Shor's algorithm shows quantum computing's potential to break RSA encryption efficiently.
Quantum Fourier transform is key to leveraging quantum computing in breaking encryption.
Implementation of quantum computing in encryption breaking could revolutionize data security.
NIST's competition seeks new cryptographic algorithms resistant to quantum computing attacks.
Lattice-based encryption algorithms emerge as strong candidates for post-quantum cryptography.
The complexity of solving the closest vector problem in high dimensions secures lattice-based encryption.
Quantum computing and AI's future role emphasizes the importance of understanding and adapting to technological advances.
Brilliant's courses on quantum algorithms and cryptography offer deep dives into the future of computing and security.
The quest for quantum-resistant encryption highlights the ongoing battle between encryption technology and computational advances.
Transcripts
Browse More Related Video
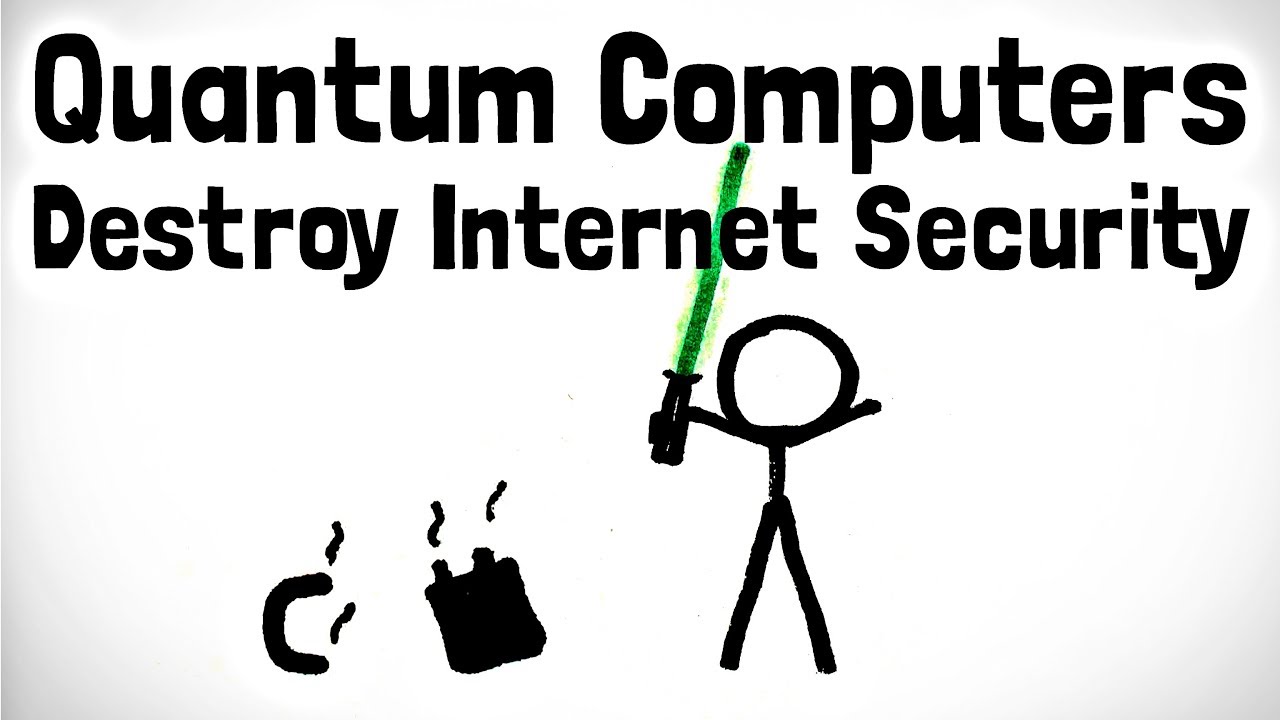
How Quantum Computers Break Encryption | Shor's Algorithm Explained
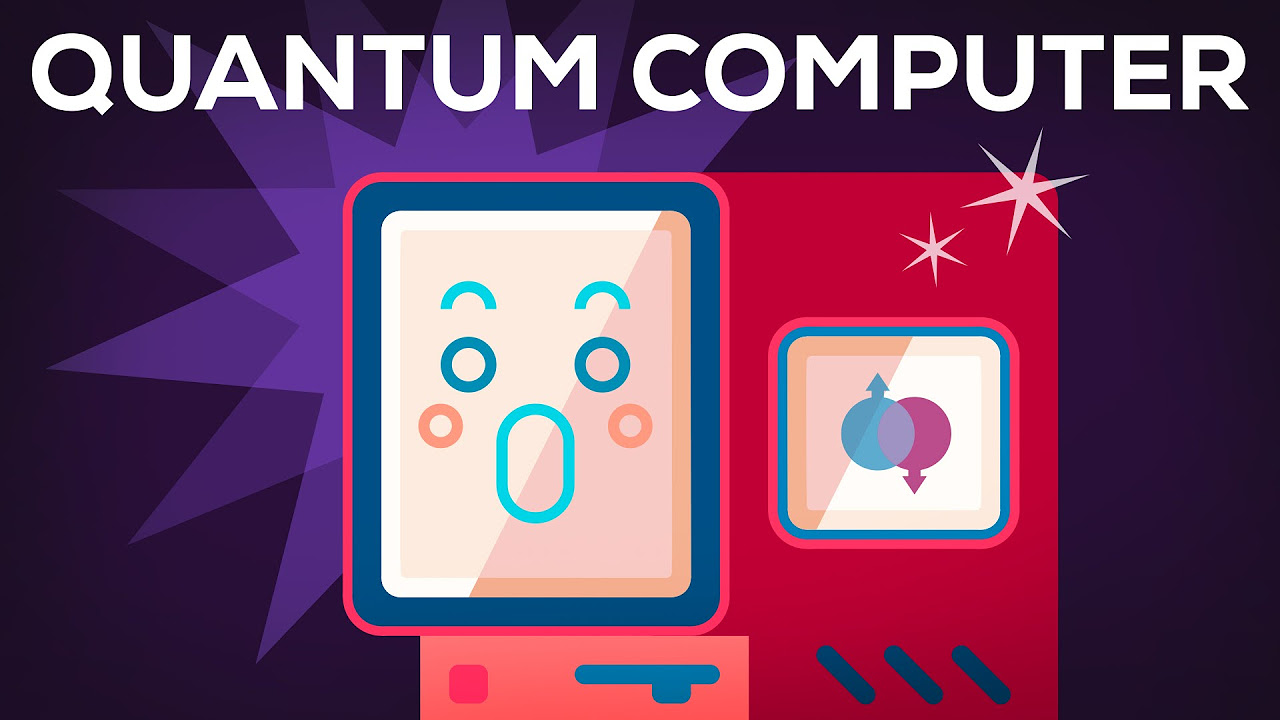
Quantum Computers Explained โ Limits of Human Technology
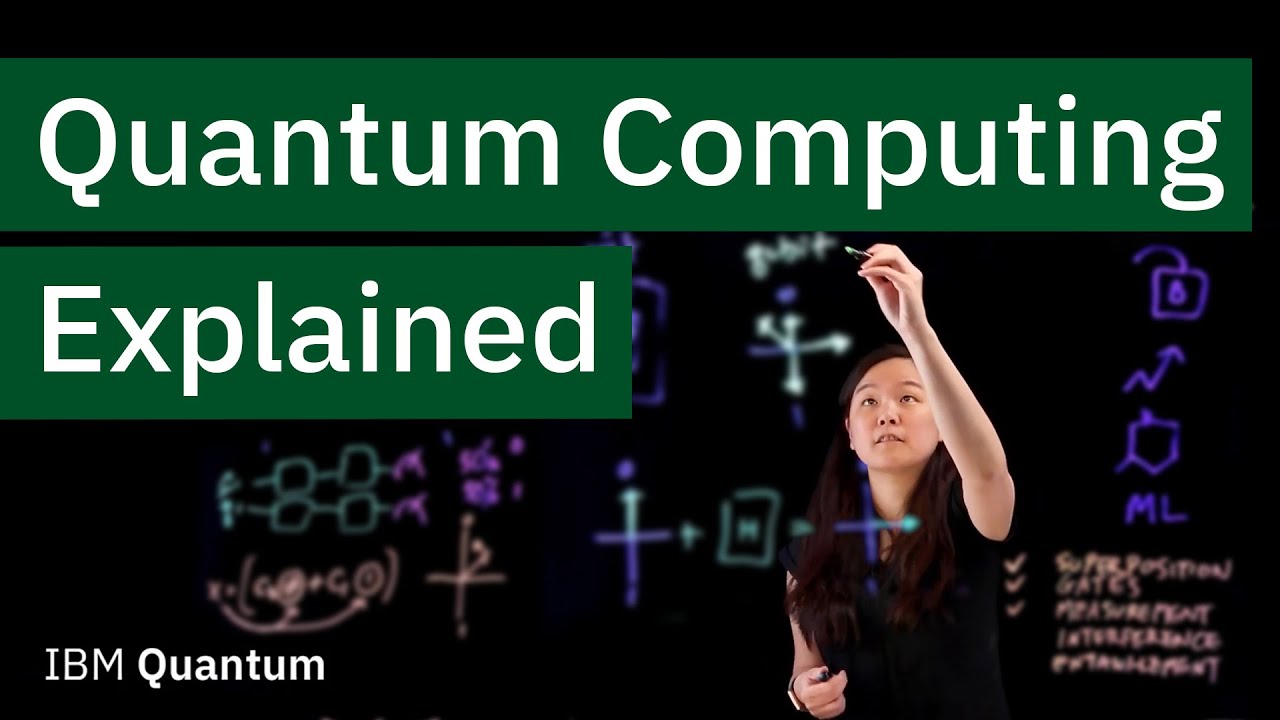
What is Quantum Computing?
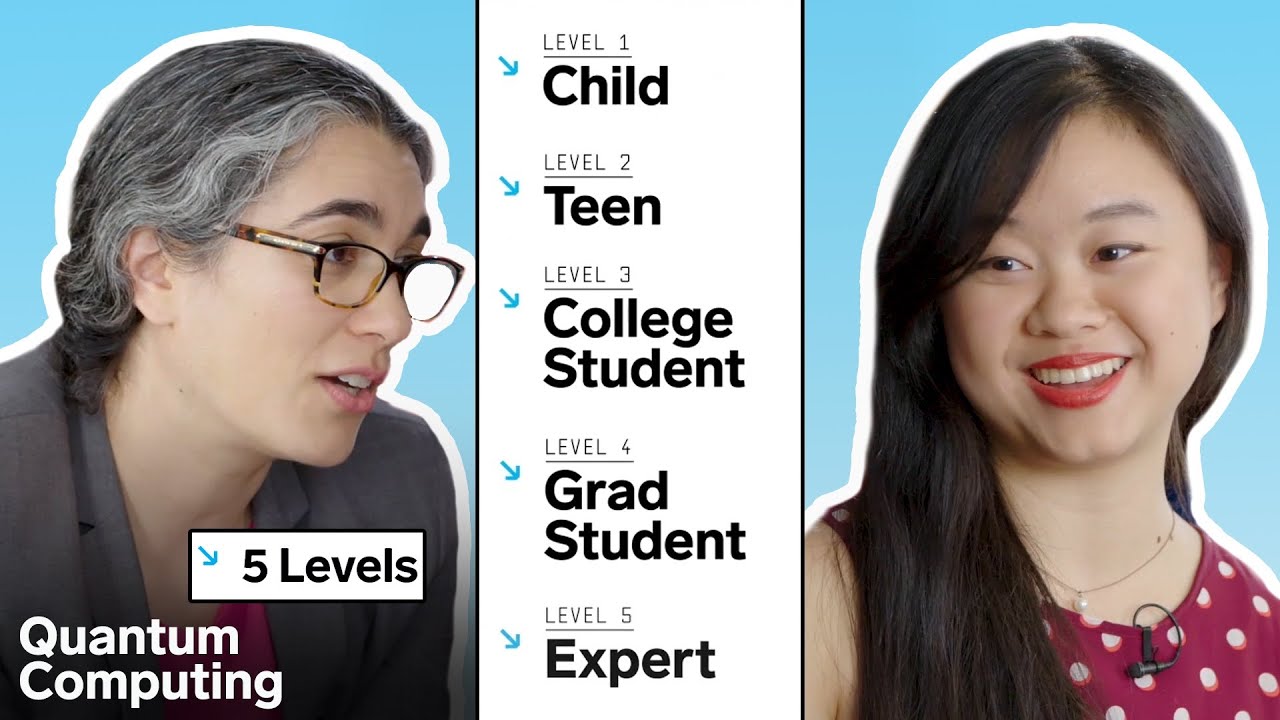
Quantum Computing Expert Explains One Concept in 5 Levels of Difficulty | WIRED
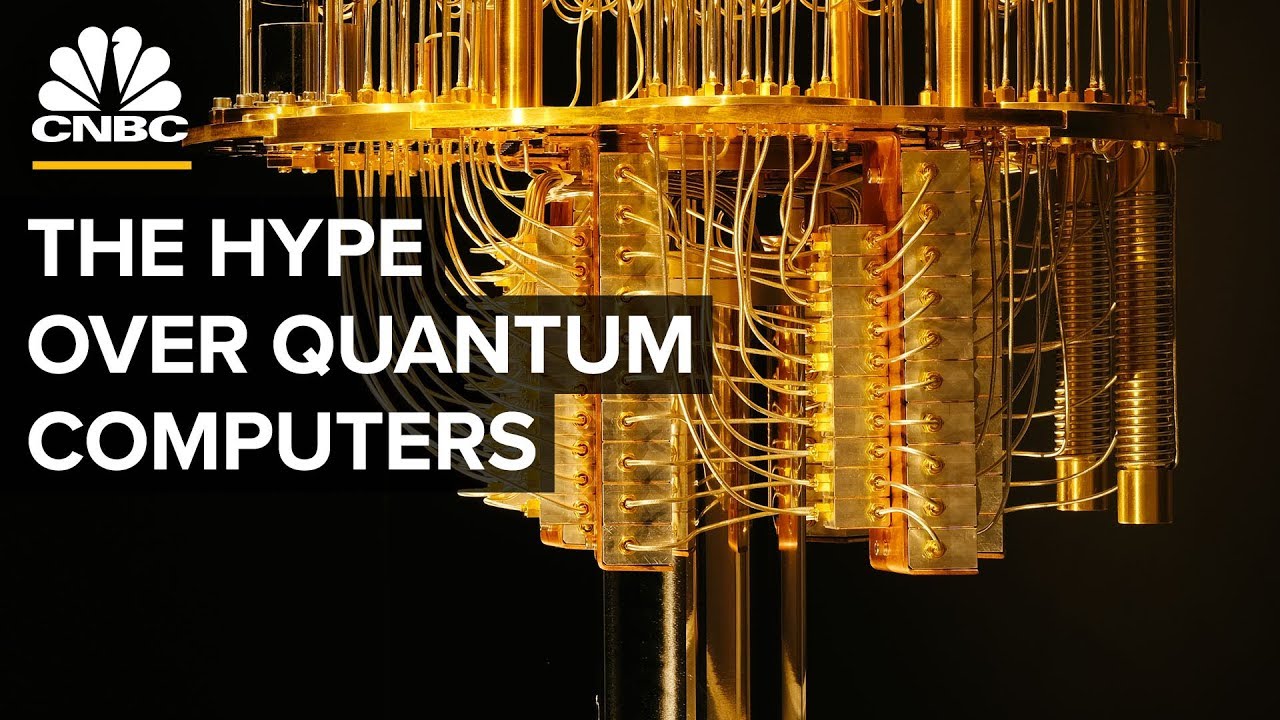
The Hype Over Quantum Computers, Explained

Quantum Computers, Explained With Quantum Physics
5.0 / 5 (0 votes)
Thanks for rating: