AP Physics 2 Magnetism and Electromagnetic Induction Review
TLDRThis video script offers a comprehensive review of magnetism in the context of AP Physics. It delves into the origins of magnetism from moving charges within particles, discusses ferromagnetic materials like iron, nickel, and cobalt, and their alignment of magnetic domains. The script explores the concept of magnetic fields, the Earth's magnetic field, and the fundamental principles governing magnetic force on moving charged particles. It also covers the relationship between electricity and magnetism, including Faraday's law of electromagnetic induction and Lenz's law, highlighting the interplay of changing magnetic flux and induced EMF. The summary encapsulates the educational content, aiming to engage viewers interested in the physics of magnetism.
Takeaways
- π The origin of magnetism lies in the motion of charged particles within atoms, creating small magnetic dipole moments that typically cancel out in most materials due to random orientation.
- π§² Ferromagnetic materials like iron, nickel, and cobalt have magnetic domains where dipole moments align, allowing the material to become magnetic when placed in an external magnetic field.
- πΊ All magnets have both a North and South Pole, and no single pole magnet has ever been observed, indicating that magnetic materials must have both poles.
- π Earth's magnetic field is such that the geographic North Pole is actually the South Magnetic Pole, as indicated by a compass needle.
- π The magnetic force on a moving charged particle depends on the charge, its velocity, the strength of the magnetic field, and the angle between the velocity and the magnetic field.
- π€ The right-hand rule is used to determine the direction of the magnetic force on a charged particle, and it's crucial when the particle's velocity is perpendicular to the magnetic field.
- π The magnetic field around a current-carrying wire can be determined using a modified right-hand rule, where the thumb points in the direction of the current and the fingers indicate the magnetic field lines around the wire.
- π A charged particle in a magnetic field experiences a centripetal force, causing it to move in a circular motion, with the radius of the circle determined by the particle's velocity, charge, mass, and the magnetic field strength.
- π Magnetic flux, denoted by Ξ¦, represents the amount of magnetic field passing through a given area and is influenced by the angle between the magnetic field and the area's orientation.
- π Faraday's law and Lenz's law describe the relationship between changing magnetic flux and the induced electromotive force (EMF), where the induced current's magnetic field opposes the change in flux.
- π Motional EMF is the potential difference induced across a conductor moving through a magnetic field, which can drive a current if the conductor is part of a closed loop.
Q & A
What causes magnetism at the atomic level?
-Magnetism at the atomic level is caused by the motion of charged particles within atoms. These moving charges create small magnetic dipole moments, resulting in a magnetic field.
Why are most materials not magnetic?
-Most materials are not magnetic because the magnetic dipole moments within them are oriented randomly, which causes them to cancel each other out and produce no measurable magnetic effect.
What are ferromagnetic materials and how do they differ from other materials?
-Ferromagnetic materials, such as iron, nickel, and cobalt, have magnetic domains where multiple dipole moments are aligned. Unlike other materials, these domains can become aligned with an external magnetic field, causing the material to become magnetic.
What happens when a ferromagnetic material is placed in an external magnetic field?
-When a ferromagnetic material is placed in an external magnetic field, the domains within the material can become aligned with the external field, resulting in the material becoming magnetic.
Why does cutting a magnet in half result in two smaller magnets, each with a North and South Pole?
-Cutting a magnet in half results in two smaller magnets because magnetic poles always come in pairs. No single pole has ever been observed, so each new piece will have both a North and South Pole.
What is the relationship between electric field lines and magnetic field lines?
-Electric field lines start and end on charges, while magnetic field lines form closed loops that go from one pole to the other and continue through the magnet, unlike electric field lines which do not form closed loops.
What is the SI unit of magnetic field strength?
-The SI unit of magnetic field strength is the Tesla.
How is the magnetic force experienced by a moving charged particle related to its charge, velocity, and the magnetic field?
-The magnetic force on a moving charged particle is given by the equation F = Q * v * B * sin(theta), where Q is the charge, v is the velocity, B is the magnetic field strength, and theta is the angle between the velocity and the magnetic field.
What is the right-hand rule for determining the direction of the magnetic force on a charged particle?
-The right-hand rule for determining the direction of the magnetic force involves pointing the fingers in the direction of the particle's velocity, curling them in the direction of the magnetic field, and the thumb will point in the direction of the force for a positive charge.
How does the motion of a charged particle in a magnetic field affect its trajectory?
-The magnetic force on a charged particle is always perpendicular to its velocity, causing the particle to move in a circular path due to the centripetal force provided by the magnetic field.
What is Faraday's law and how is it related to magnetic flux?
-Faraday's law states that a changing magnetic flux through a closed loop induces an electromotive force (EMF) in the loop. This is related to magnetic flux as the change in flux over time is what causes the induction of the EMF.
What is Lenz's law and how does it apply to a magnet falling through a conducting pipe?
-Lenz's law states that the induced EMF and resulting current from a changing magnetic flux will create a magnetic field that opposes the change in flux. In the case of a magnet falling through a conducting pipe, the induced current creates a magnetic field that opposes the fall, slowing the magnet down.
What is motional EMF and how is it generated?
-Motional EMF is the electromotive force induced across a conductor when it moves through a magnetic field. It is generated due to the relative motion between the conductor and the magnetic field, causing a separation of charges within the conductor.
How does the current direction change when a conductor moves into and out of a magnetic field?
-When a conductor moves into a magnetic field, the current flows in one direction, and when it moves out, the current flows in the opposite direction due to the change in the direction of the magnetic force acting on the charges within the conductor.
Outlines
π§² Origins and Basics of Magnetism
This paragraph delves into the fundamental concepts of magnetism, starting with its origins from moving charged particles within atoms. It explains how these particles create small magnetic dipole moments, which are usually randomized in most materials, cancelling each other out. However, in ferromagnetic materials like iron, nickel, and cobalt, these dipoles can align within magnetic domains, responding to external magnetic fields. The script also touches on the permanence of magnetic poles, the impossibility of isolating a single pole, and the concept of magnetic field lines, which differ from electric field lines in that they form closed loops.
π Earth's Magnetic Field and Magnetic Force
The second paragraph discusses Earth's magnetic field, clarifying the confusion between geographic and magnetic poles using a compass example. It then explores the concept of magnetic force, emphasizing the necessity for a charged particle to be in motion to experience this force. The force is described by the equation F = QvB sin(ΞΈ), where Q is the charge, v is the velocity, B is the magnetic field strength, and ΞΈ is the angle between the velocity and the magnetic field. The paragraph also explains the right-hand rule for determining the direction of the magnetic force on a moving charged particle and introduces the SI unit of magnetic field strength, the Tesla.
π The Dynamics of Charged Particles in Magnetic Fields
This section examines the behavior of charged particles when subjected to magnetic forces. It explains that these forces are always perpendicular to the particle's velocity, resulting in circular motion. The centripetal force causing this motion is identified as the magnetic force, and the relationship between the radius of the circular path (R), the velocity (V), and the magnetic field strength is established using Newton's second law. The significance of this relationship for determining the radius, velocity, and mass of the particle is highlighted.
π€οΈ Magnetic Effects on Current-Carrying Wires
The fourth paragraph investigates the impact of magnetic fields on current-carrying wires. It presents the formula for the magnetic force experienced by a wire in a magnetic field, emphasizing the dependence on current, wire length, magnetic field strength, and the angle between the wire and the field. Additionally, it introduces the concept of magnetic fields generated by the current in the wire itself, using a modified right-hand rule to determine the direction of this field. The paragraph concludes with Ampère's law, which describes the magnetic field strength around a wire in relation to the current and distance from the wire.
π Magnetic Flux and Faraday's Law of Electromagnetic Induction
The script introduces the concept of magnetic flux, defined as the rate of magnetic field flow through a surface. It explains how flux is maximized when the magnetic field is parallel to the surface and minimized when it is perpendicular. The paragraph then connects changing magnetic flux to Faraday's law of electromagnetic induction, which states that a changing flux induces an electromotive force (EMF) in a closed circuit. The relationship between electricity and magnetism is further explored through examples of how motion and orientation affect the generation of EMF and current.
π Lenz's Law and Conservation of Energy in Electromagnetic Induction
This section delves into Lenz's law, which describes the direction of the induced EMF and current in response to a changing magnetic flux. It states that the induced magnetic field will oppose the change in flux, a concept demonstrated through the example of a magnet falling through a conductive pipe, causing the magnet to slow down. The conservation of energy principle is used to explain this phenomenon, where the energy lost by the falling magnet is transferred to the induced current in the pipe. The paragraph also revisits motional EMF, which occurs when a conductor moves through a magnetic field, generating a potential difference and potentially a current if a closed loop is present.
π Practical Examples of Electromagnetic Induction
The final paragraph provides practical examples of electromagnetic induction, such as a cart with a wire loop moving through a magnetic field. It explains how the motion of the conductor through the field induces a potential difference and current in specific conditions. The paragraph details the direction of the induced current as the cart enters and leaves the magnetic field and why no current flows while the loop is entirely within the field due to opposing currents canceling each other out. The summary emphasizes the relationship between the speed of the conductor and the induced EMF, highlighting the practical applications of these principles.
Mindmap
Keywords
π‘Magnetism
π‘Magnetic Dipole Moment
π‘Ferromagnetic Materials
π‘Magnetic Domains
π‘Magnetic Field
π‘Magnetic Field Lines
π‘Magnetic Force
π‘Right-Hand Rule
π‘Magnetic Flux
π‘Faraday's Law
π‘Lenz's Law
π‘Motional EMF
Highlights
Magnetism originates from moving charged particles within atoms, creating small magnetic dipole moments.
Most materials are non-magnetic due to the random orientation of their dipole moments, cancelling out magnetic effects.
Ferromagnetic materials like iron, nickel, and cobalt have magnetic domains where dipole moments align, responding to external magnetic fields.
External magnetic fields can align domains in ferromagnetic materials, inducing magnetism.
Magnet cutting demonstrates that each piece retains both poles, emphasizing the intrinsic link between North and South poles.
Magnetic field lines are loops, contrasting with electric field lines, and are indicative of the force experienced by magnetic materials.
Earth's magnetic field is highlighted, with the geographic North Pole being the magnetic South Pole.
Magnetic force on a moving charged particle is dependent on charge, velocity, magnetic field strength, and the angle between them.
The right-hand rule is introduced to determine the direction of the magnetic force on a charged particle.
Magnetic forces are always exerted at right angles to the velocity, causing charged particles to move in circular paths.
The relationship between the radius of the circular path, velocity, and mass of a charged particle in a magnetic field is discussed.
Current-carrying wires experience magnetic forces, with the force dependent on current, wire length in the field, and the angle with the field.
The magnetic field around a wire is proportional to the current and inversely proportional to the distance from the wire.
Magnetic flux is defined as the rate of magnetic field flow through an area, with its maximum when the field aligns with the area.
Faraday's law connects changing magnetic flux through a coil to the induced EMF, demonstrating the link between magnetism and electricity.
Lenz's law is explained, stating that induced EMF and current oppose changes in magnetic flux, as illustrated by a magnet falling through a conductor pipe.
Motional EMF is described, resulting from a conductor moving through a magnetic field, inducing a potential difference and potentially a current.
The effect of a moving conductor through a magnetic field is detailed, with current generation occurring only upon entry or exit, not while fully within the field.
Transcripts
Browse More Related Video

2022 Live Review 5 | AP Physics 2 | Practicing Key Concepts in Magnetism with FRQ's
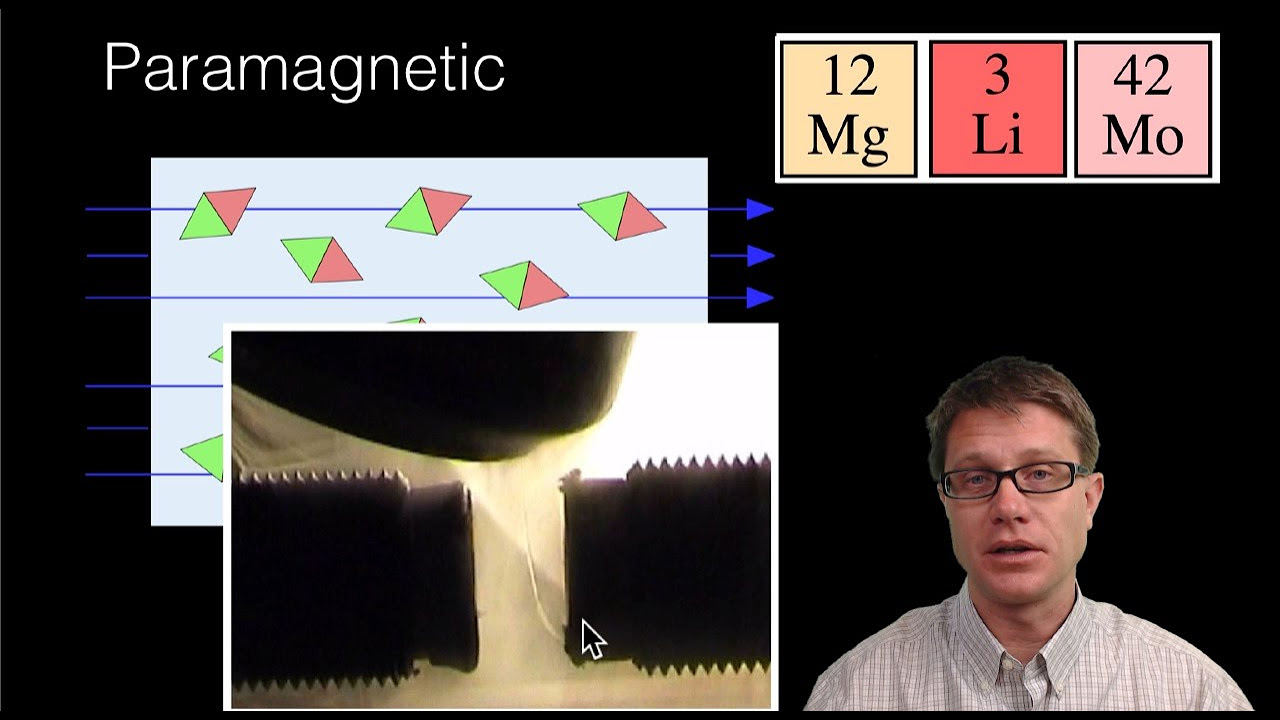
Magnetic Properties
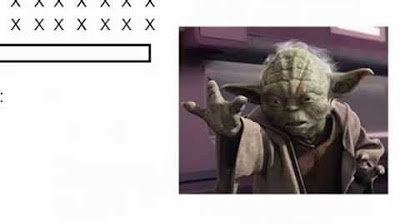
AP Physics B Exam Review - Electricity and Magnetism

Magnetism, Magnetic Field Force, Right Hand Rule, Ampere's Law, Torque, Solenoid, Physics Problems
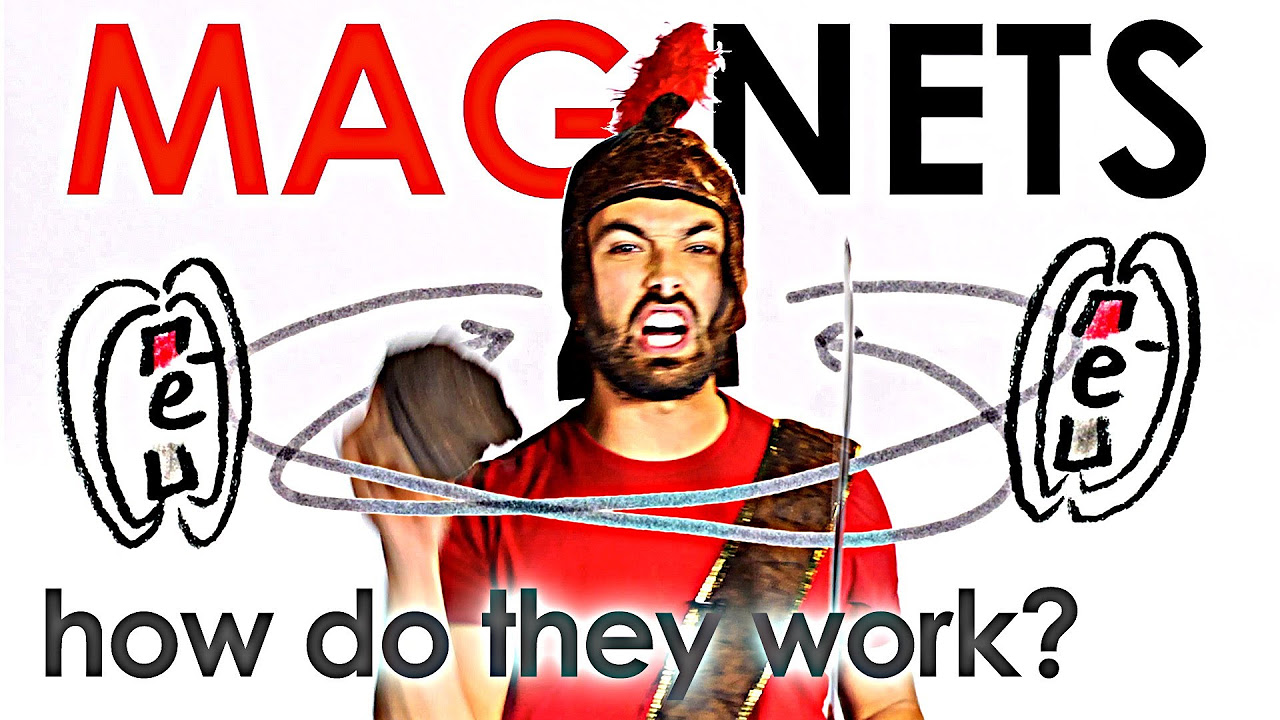
MAGNETS: How Do They Work?
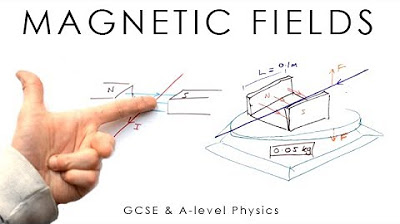
Magnetic Fields, Flux Density & Motor Effect - GCSE & A-level Physics (full version)
5.0 / 5 (0 votes)
Thanks for rating: