Physics - Ch 66 Ch 4 Quantum Mechanics: Schrodinger(47 of 92) Why solid Not Radiate like Oscillator?
TLDRThe video script discusses the behavior of atoms within a solid compared to a quantum mechanical harmonic oscillator. It explains that while individual atoms oscillate at specific frequencies when isolated, in a solid, the intermolecular forces and thermal energy lead to a complex, random vibrational pattern. This results in a wide range of frequencies and a blackbody radiation curve that differs significantly from that of a single quantum oscillator. The script highlights the discrepancy between the expected quantum states and the actual behavior of atoms in a solid at various temperatures, leading to a diverse array of emitted wavelengths and frequencies.
Takeaways
- π΅ Each atom in a harmonic oscillator behaves like a quantum mechanical oscillator, oscillating at a specific frequency.
- π‘οΈ The radiation from a solid is not at a specific frequency of the oscillator's vibration, but rather depends on its temperature, following the Wien's displacement law.
- π The predominant wavelength emitted by an object at a certain temperature can be calculated using Wien's displacement law (Ξ»max = b / T), where b is Wien's displacement constant.
- π When the temperature of a solid increases, the predominant wavelengths of radiation change, which contradicts the behavior of a quantum oscillator whose frequency is fixed by its mass and spring constant.
- π The atoms in a solid do not behave like independent quantum mechanical oscillators due to the intermolecular forces and interactions between them.
- π₯ The vibrations of atoms in a solid are random and not confined to a single frequency, leading to a wide spread of wavelengths and frequencies in the emitted radiation.
- π The complex interactions among a vast number of atoms in a solid result in a variety of vibrational frequencies, unlike the single frequency expected from a simple quantum oscillator.
- π At higher temperatures, the range of wavelengths emitted by a solid broadens, with frequencies varying significantly from the predictions of a single quantum oscillator.
- π The collective behavior of atoms in a solid, influenced by their environment and interactions, leads to a blackbody radiation curve that is distinct from the radiation of a single quantum oscillator.
- π« The solid's radiation curve is not consistent with the quantum mechanical radiation curve of a single oscillator due to the complex, interconnected nature of atomic vibrations in the solid.
Q & A
What is a quantum mechanical harmonic oscillator?
-A quantum mechanical harmonic oscillator is a system where each atom oscillates at a particular frequency, and the energy gained by absorbing a photon results in a larger amplitude of oscillation, not an increase in the frequency of oscillation.
How does the radiation from a solid differ from the radiation according to the specific frequency of an oscillator?
-The radiation from a solid is a function of its temperature rather than the specific frequency of the vibration of an oscillator. It does not follow the quantum mechanical harmonic oscillator model, which would have radiation at a specific frequency determined by the oscillator's quantum states.
What is the Wien's displacement law and how is it used to determine the temperature of an object?
-The Wien's displacement law states that the predominant wavelength of radiation emitted by an object at a particular temperature can be defined by a constant (0.0000929 meters times Kelvin) divided by the temperature of the object. By observing the predominant wavelength of radiation, one can calculate the temperature of the object.
Why doesn't a solid behave like a quantum mechanical oscillator when its temperature is increased?
-When the temperature of a solid is increased, the atoms within the solid do not oscillate at a single frequency like a quantum mechanical oscillator. Instead, the atoms vibrate in random directions and at various frequencies due to intermolecular forces and thermal energy, resulting in a wide range of frequencies and a different radiation pattern.
How does the angular frequency of oscillation relate to the mass and spring constant in a quantum mechanical harmonic oscillator?
-The angular frequency of oscillation in a quantum mechanical harmonic oscillator is determined by the mass of the oscillating particle and the spring constant. It is given by the formula β(spring constant / mass), and it remains fixed for a given oscillator, independent of the energy absorbed or released.
What is the relationship between wavelength and frequency for a photon?
-The relationship between the wavelength and frequency of a photon is given by the equation where wavelength equals the speed of light divided by the frequency. This is derived from the fact that the speed of light is constant, and thus the product of wavelength and frequency for a given photon must also be constant.
How does the radiation curve of a solid at different temperatures illustrate the behavior of atoms within the solid?
-The radiation curve of a solid at different temperatures shows a wide spread of wavelengths and frequencies, indicating that the atoms within the solid are not oscillating in a simple, predictable manner like a quantum mechanical harmonic oscillator. Instead, they exhibit a range of vibrational frequencies due to the complex interactions and thermal energy.
What is the significance of the wide range of frequencies observed in the radiation from a solid?
-The wide range of frequencies in the radiation from a solid indicates that the atoms are not behaving like independent quantum mechanical oscillators. Instead, their vibrations are influenced by the interactions with other atoms and the thermal energy, leading to a complex pattern of oscillations at various frequencies.
How does the behavior of atoms in a solid differ from the behavior of atoms in a simple two-atom system?
-In a solid, atoms are part of a complex network of intermolecular forces and their vibrations are influenced by the entire structure. This results in a wide range of oscillation frequencies and directions, unlike a simple two-atom system where the oscillations are more predictable and controlled by a single spring-like force.
What is the implication of the different radiation curves for a solid compared to a single quantum mechanical oscillator?
-The different radiation curves for a solid compared to a single quantum mechanical oscillator imply that solids cannot be accurately modeled as simple quantum mechanical oscillators. The complex interactions between atoms and the influence of temperature result in a broader spectrum of radiation that does not follow the discrete energy levels of quantum states.
Outlines
π Quantum Mechanics and Oscillation Frequencies
This paragraph discusses the concept of a quantum mechanical harmonic oscillator and its application to atoms within a solid. It explains that each atom oscillates at a specific frequency and energy, and that the absorption of a photon results in a larger amplitude of oscillation, not an increase in frequency. The paragraph then poses the question of why solids do not behave like quantum mechanical oscillators, noting that the radiation emitted from a solid is temperature-dependent and not at a specific frequency as predicted by the oscillator model. The Wien's displacement law is introduced to illustrate how the predominant wavelength of radiation from an object can be determined by its temperature. However, the paragraph highlights the discrepancy between the expected quantum mechanical behavior and the actual behavior observed in solids at various temperatures, indicating that the atoms in a solid do not behave as independent quantum oscillators.
π₯ Solids vs. Quantum Oscillators: Frequency and Temperature
The second paragraph delves into the behavior of atoms in a solid at different temperatures and how it contrasts with the expectations of a quantum mechanical oscillator. It explains that the intermolecular forces and bonds within a solid cause vibrations to ripple through the atoms, leading to a random motion rather than a simple oscillatory pattern. As the temperature increases, the vibrations become more chaotic, resulting in a wide range of frequencies and a blackbody radiation curve that differs significantly from the radiation curve of a single quantum oscillator. The paragraph concludes by emphasizing that the collective behavior of atoms in a solid, influenced by their interactions with each other, results in a complex radiation pattern that cannot be described by the simple model of a quantum mechanical oscillator.
Mindmap
Keywords
π‘Harmonic Oscillator
π‘Quantum Mechanic Oscillator
π‘Photon
π‘Wien's Displacement Law
π‘Blackbody Radiation
π‘Temperature
π‘Angular Frequency
π‘Intermolecular Forces
π‘Wavelength
π‘Radiation
π‘Frequency
Highlights
Each atom within a harmonic oscillator acts as a quantum mechanic oscillator, oscillating at a particular frequency.
Energy gained by absorbing a photon results in a larger amplitude of oscillation, not an increase in frequency.
Solids do not behave like quantum mechanic oscillators, as their radiation is not according to the specific frequency of the oscillator's vibration.
The radiation from a solid is a function of its temperature, following the Wien's displacement law.
The predominant wavelength from an object at a particular temperature can be defined by Wien's displacement law (Ξ»max = b / T).
For a typical spring constant and atomic mass, the angular frequency of oscillation is fixed for a quantum oscillator.
The behavior of atoms in a solid contradicts the fixed angular frequency expected in a quantum oscillator when the temperature changes.
Black body radiation curves show that as temperature increases, the predominant wavelengths emitted by a solid change significantly.
At higher temperatures, the range of wavelengths emitted by a solid spans from 100 nanometers to over 2000 nanometers.
The frequencies of radiation from a solid at different temperatures are very different from the frequencies expected from a single quantum mechanic oscillator.
Atoms inside a solid are interconnected and their vibrations are influenced by intermolecular forces and bonds.
As thermal energy increases, the vibrations of atoms in a solid become more random and less like simple oscillatory motion.
The wide range of frequencies observed in the radiation from a solid at any given temperature indicates that individual atoms do not behave like isolated quantum oscillators.
The radiation curve of a solid is very different from the quantum mechanic radiation curve of a single oscillator at quantum mechanic states.
The complex interplay of vibrations and energies among a vast number of atoms in a solid leads to the observed wide range of radiation wavelengths.
The behavior of a solid when heated is a result of the collective and interconnected vibrations of its constituent atoms, not simple quantum oscillations.
The wide array of frequency ranges in a solid's radiation is due to the complex interactions among atoms, rather than isolated quantum states.
Transcripts
Browse More Related Video
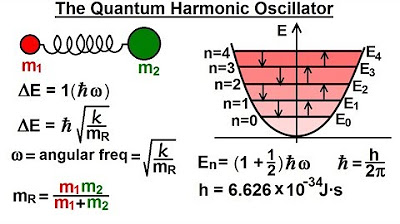
Physics - Ch 66 Ch 4 Quantum Mechanics: Schrodinger Eqn (39 of 92) What is the Quantum Oscillator?
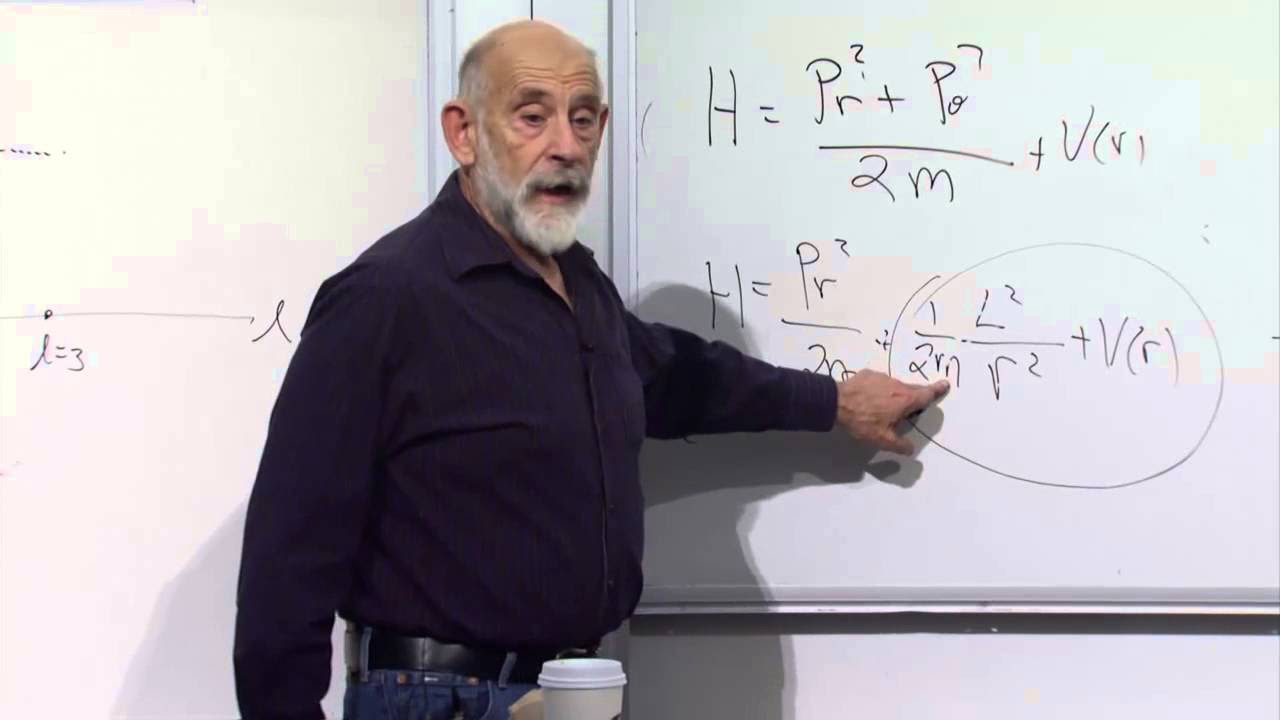
Advanced Quantum Mechanics Lecture 3

Physics - Ch 66 Ch 4 Quantum Mechanics: Schrodinger Eqn (46 of 92) Quantum Nature of Oscillator 2

Physics - Ch 66 Ch 4 Quantum Mechanics: Schrodinger Eqn (51 of 92) Oscillator Amplitude - Diatomic

Physics - Ch 66 Ch 4 Quantum Mechanics: Schrodinger Eqn (48 of 92) How Oscillator Increases energy

Advanced Quantum Mechanics Lecture 6
5.0 / 5 (0 votes)
Thanks for rating: