Nelly Ng: The fundamental limits of efficiency for quantum heat engines
TLDRThis lecture delves into the realm of quantum thermodynamics, exploring the theoretical limits of efficiency for quantum heat engines. The speaker, from Phylum University Berlin, discusses the application of information theory to thermodynamics, the concept of work extraction in quantum systems, and the challenges in defining work in quantum thermodynamics. The talk also covers the use of resource theories to model heat engines, the conditions for work extraction, and the surprising finding that certain quantum heat engines can exceed the traditional Carnot efficiency under specific conditions.
Takeaways
- ๐ฌ The speaker from Phylum University Berlin discusses the fundamental efficiency limits for quantum heat engines within the context of quantum thermodynamics.
- ๐ The talk emphasizes the importance of information theory and its application to describe quantum thermodynamics, particularly in the study of heat engines and their theoretical limits.
- ๐ค The research is a collaborative effort with Lucia Woods from Zurich and the speaker's former PhD supervisor Stephanie.
- ๐ Classical thermodynamics is contrasted with quantum thermodynamics, highlighting the differences in how work and heat are treated, especially in small-scale quantum systems.
- ๐งฌ The speaker mentions mesoscopic thermodynamics, which deals with systems that are small enough to exhibit quantum effects but are still effectively classical.
- ๐ The concept of work extraction in quantum thermodynamics is complex due to the potential for energy fluctuations to be comparable to the work stored in quantum systems.
- ๐ The speaker provides examples of quantum heat engines, such as a quantum refrigerator and a trapped ion system, to illustrate the practical applications of theoretical concepts.
- ๐ค The definition of work in quantum thermodynamics is a topic of debate, with different perspectives and definitions being discussed, including single-shot work and the use of resource theories.
- ๐ The script delves into the resource theory framework, explaining how it can be used to model and study heat engines, taking into account different types of work and their efficiencies.
- ๐ The analysis of heat engines using resource theory reveals that perfect work extraction is not possible under certain conditions, while near-perfect work can sometimes achieve Carnot efficiency.
- ๐ฎ The speaker concludes by highlighting the importance of clearly defining work in any heat engine analysis and suggests that further research could bridge the gap between theoretical models and experimental setups.
Q & A
What is the main topic of the speaker's presentation?
-The main topic of the presentation is the study of quantum heat engines and the theoretical limits on their achievable efficiency within the framework of quantum thermodynamics.
What is the significance of the research on quantum heat engines?
-The significance lies in understanding the fundamental efficiency limits of quantum heat engines, which can provide insights into the performance of quantum mechanical systems used in various technologies such as quantum computation.
What is the role of information theory in the study of quantum heat engines?
-Information theory provides a new framework to describe and analyze quantum systems, including heat engines, by offering tools to evaluate the theoretical limits of work extraction and efficiency.
What is the difference between classical thermodynamics and the mesoscopic regime discussed in the script?
-Classical thermodynamics is applied to macroscopic systems in equilibrium, while the mesoscopic regime deals with smaller, non-equilibrium systems where concepts like work extraction become associated with stochastic processes.
How does the definition of work differ in quantum thermodynamics compared to classical thermodynamics?
-In quantum thermodynamics, the definition of work is less clear-cut due to the small energy scales comparable to thermal fluctuations, making the distinction between heat and work less distinct.
What are some examples of quantum mechanical systems that can be considered as quantum heat engines?
-Examples include a theoretical quantum refrigerator with a finite size machine containing a single electron, and a trapped ion interacting with laser beams and electric noise, which can be used to extract work and fuel mechanical motion.
What is the concept of 'coherence' in quantum thermodynamics?
-Coherence refers to the quantum effect where energy levels can build up phase relations with each other, which can be a factor in the efficiency of quantum heat engines.
What is the role of a catalyst in the context of quantum thermodynamics and heat engines?
-A catalyst in quantum thermodynamics can be a finite-dimensional quantum state that is used in a process and must be returned to its original state, allowing for more flexible state transformations while preserving certain properties.
What is the 'quasi-static limit' in the context of heat engines?
-The quasi-static limit refers to a scenario where the final state of the cold bath has a well-defined temperature very close to the initial temperature, allowing for the analysis of maximum achievable efficiency.
What is the significance of the Carnot efficiency in the context of quantum heat engines?
-The Carnot efficiency is an upper bound on the efficiency that a heat engine can achieve, and it serves as a benchmark to evaluate the performance of quantum heat engines within the resource theory framework.
How does the speaker's analysis of quantum heat engines relate to experimental settings?
-The speaker acknowledges a disconnect between the theoretical framework of resource theory and experimental settings, suggesting a need for future work to bridge the gap and apply these results to practical scenarios.
Outlines
๐ Introduction to Quantum Thermodynamics
The speaker from the University of Berlin is introduced to discuss quantum thermodynamics, specifically focusing on the theoretical limits of efficiency for quantum heat engines. The talk is set against the backdrop of a conference that has extensively covered information theory and its applications in thermodynamics. The speaker outlines the joint work with Lucia Woods and Stephanie, emphasizing the evolution of thermodynamics from macroscopic systems to the mesoscopic regime, which includes non-equilibrium processes. The goal is to explore the fundamental efficiency limits of quantum heat engines within this framework.
๐ Quantum Thermodynamics and its Experimental Relevance
The speaker delves into the essence of quantum thermodynamics, highlighting the unique features of quantum systems such as small size, finite energy dimensions, and the presence of quantum effects like coherence and entanglement. The talk also provides concrete examples of quantum machines that experimentalists are interested in, such as a quantum refrigerator and a trapped ion system, to illustrate the practical applications of quantum thermodynamics. The speaker emphasizes the importance of understanding the efficiency of quantum heat engines in the context of quantum computation and other quantum technologies.
๐ง Theoretical Framework for Quantum Heat Engines
The speaker presents a theoretical framework for analyzing quantum heat engines, starting with a discussion on the conditions and mechanisms for quantum states to equilibrate. The focus then shifts to the energy cost of computations and information processing, the role of coherence and entanglement in quantum systems, and the usefulness of quantum heat engines. The speaker aims to apply the resource steering framework to construct a generic model of quantum heat engines and evaluate their theoretical efficiency limits.
๐ Defining Work in Quantum Thermodynamics
A significant portion of the talk is dedicated to the challenge of defining work in quantum thermodynamics. The speaker discusses various perspectives, including the information theory approach, the axiomatic approach, and the use of resource theories to quantify work. The talk also addresses the difficulty in distinguishing between heat and work in quantum systems due to their small energy scales and the presence of thermal fluctuations, leading to a range of different classifications for work extraction in the quantum regime.
๐ The Role of Catalysis in Quantum Processes
The concept of catalysis in quantum thermodynamics is explored, with the speaker explaining how catalysts can be used to facilitate state transitions in quantum systems. The talk differentiates between exact catalysis, where the catalyst returns to its original state without any correlation with the system, and inexact catalysis, which allows for small errors in the final state of the catalyst. The speaker also discusses the implications of these concepts for the implementation of quantum processes in experimental settings.
๐ Analysis of Quantum Heat Engines with Catalysis
The speaker provides an in-depth analysis of quantum heat engines within the framework of catalysis, discussing how to apply resource theories to build and study these engines. The focus is on using a cold bath as a system of interest and attaching a battery to extract work. The speaker outlines the assumptions of the setup, including the initial state of the systems and the use of catalytic operations to achieve work extraction.
๐ Maximizing Efficiency in Quantum Heat Engines
The talk moves towards understanding the maximum achievable efficiency of quantum heat engines. The speaker discusses the use of free energy and generalized free energies as constraints to determine the efficiency limits. The analysis reveals that the Carnot efficiency serves as an upper bound for the efficiency that can be achieved under certain conditions, such as the quasi-static limit, which is defined within the resource theory framework.
๐ Perfect and Near-Perfect Work in Quantum Engines
The speaker distinguishes between perfect work, where the increase in entropy of the battery is zero, and near-perfect work, which allows for a small increase in entropy. The analysis shows that while perfect work is not possible under the generalized second laws, near-perfect work can sometimes achieve the Carnot efficiency. The speaker also discusses the conditions under which these efficiencies can be achieved, highlighting the importance of the initial and final states of the cold bath.
๐ The Impact of Work Definitions on Efficiency
The talk concludes with a discussion on the impact of different work definitions on the efficiency of quantum heat engines. The speaker emphasizes that the definition of work is crucial, as it can lead to different interpretations of efficiency, especially when considering imperfect work. The speaker also presents examples where the efficiency can exceed the Carnot efficiency under certain conditions, suggesting that the understanding of work in quantum thermodynamics may need further refinement.
๐ค Open Questions and Future Directions
The speaker acknowledges open questions and suggests future directions for research in quantum thermodynamics. There is a call for a clearer understanding of work in quantum systems and the exploration of more realistic thermodynamical models. The speaker also highlights the need to bridge the gap between theoretical work and experimental practice, encouraging further research to connect the resource theory framework with experimental scenarios in quantum thermodynamics.
๐ค Audience Q&A and Discussion
The final part of the script includes a question and answer session with the audience. The speaker addresses queries related to the framework of quantum thermodynamics, the realistic scenarios where these results could apply, and the implications of different work definitions on the efficiency of quantum heat engines. The discussion delves into the nuances of the resource theory approach and its connection to experimental settings, reflecting the ongoing dialogue between theory and practice in the field of quantum thermodynamics.
Mindmap
Keywords
๐กQuantum Thermodynamics
๐กHeat Engines
๐กResource Theory
๐กEfficiency
๐กCarnot Efficiency
๐กWork Extraction
๐กQuantum Information Theory
๐กCoherence
๐กEntanglement
๐กMesoscopic Thermodynamics
๐กStatistical Physics
Highlights
Introduction of a speaker from the University of Berlin discussing quantum thermodynamics and the efficiency of quantum heat engines.
The talk covers the application of information theory to thermodynamics, providing a new framework for understanding quantum systems.
Exploration of the theoretical limits on the achievable efficiency of quantum heat engines.
Joint work with Lucia Woods and Stephanie Wehner on the fundamentals of quantum thermodynamics.
Discussion on the evolution of thermodynamics from macroscopic to mesoscopic and now to quantum regimes.
The importance of understanding non-equilibrium processes in quantum thermodynamics, such as protein folding and molecular motors.
The challenge of defining work in quantum systems due to the comparability of work storage energy to thermal fluctuations.
Different perspectives on defining work in quantum thermodynamics, including single-shot work and the use of resource theories.
The use of quantum information theory tools to revisit classical thermodynamics statements.
Key features differentiating quantum systems in thermodynamics, such as small system sizes and quantum effects like coherences and entanglement.
Examples of quantum heat engines and refrigerators, including a theoretical proposal for a quantum refrigerator.
The role of heat engines in the historical development of thermodynamics and the quest for high-efficiency designs.
The application of the resource steering framework to construct a generic model of quantum heat engines.
Analysis of the conditions and mechanisms for quantum states to equilibrate and the energy cost of computations in quantum systems.
The significance of the quasi-static limit in achieving maximum efficiency in quantum heat engines.
The potential for exceeding Carnot efficiency in certain quantum heat engine models under specific conditions.
The importance of the initial and final states of quantum systems in determining the efficiency of heat engines.
The disconnect between resource theory priorities and experimental quantum thermodynamics, suggesting a need for closer integration.
Transcripts
Browse More Related Video
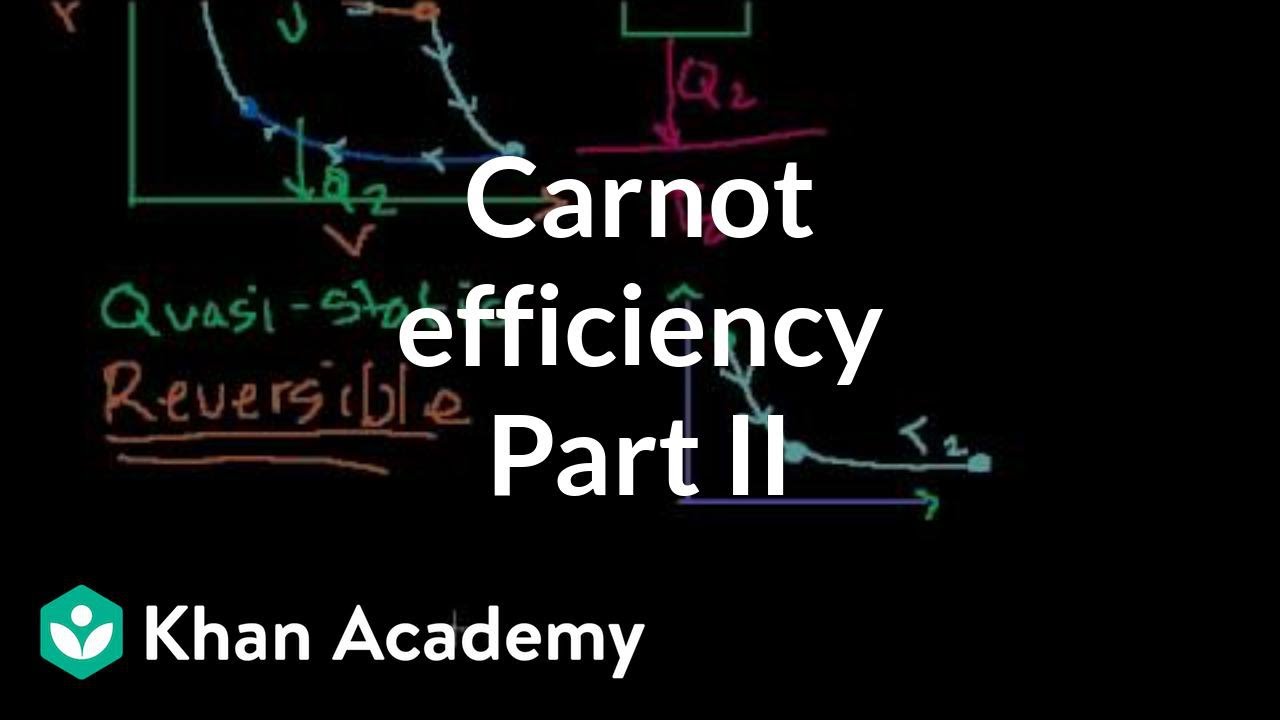
Carnot efficiency 2: Reversing the cycle | Thermodynamics | Physics | Khan Academy
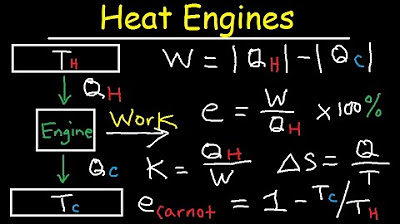
Carnot Heat Engines, Efficiency, Refrigerators, Pumps, Entropy, Thermodynamics - Second Law, Physics

Engines: Crash Course Physics #24

Heat Engines, Thermal Efficiency, & Energy Flow Diagrams - Thermodynamics & Physics Problems
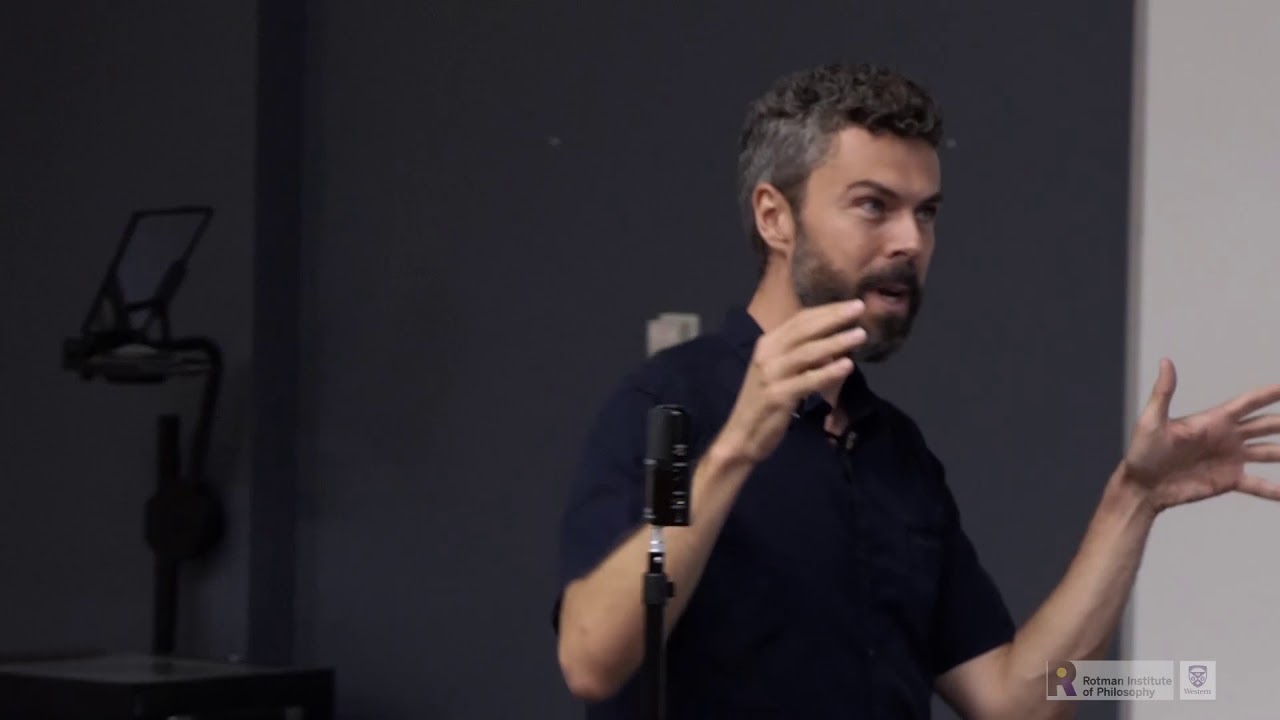
Markus Mรผller: Exact operational interpretation of the free energy without the thermodynamic limit

Carnot Engine
5.0 / 5 (0 votes)
Thanks for rating: