Bioorthogonal Chemistry, From Basic Science to Clinical Translation β Carolyn Bertozzi
TLDRIn this engaging talk, the speaker delves into the fascinating world of bioorthogonal chemistry, a field that allows for chemical reactions to occur within biological systems without interfering with natural biological processes. Starting from its origins at Berkeley to its current applications in the biopharma industry, the speaker highlights the development of foundational bioorthogonal reactions and their impact on glycoscience, drug delivery, and diagnostic imaging. The narrative is enriched with personal anecdotes and insights into the translational potential of these chemistries, emphasizing the role of curiosity and innovation in scientific discovery.
Takeaways
- π The speaker discusses the backstory of bioorthogonal chemistry, highlighting its roots in Berkeley and its evolution from basic science to translational applications.
- π§ͺ Bioorthogonal chemistry is defined as chemical reactions that do not interact with or interfere with biological systems, allowing for selective reactions within a living organism.
- π The speaker's interest in glycoscience, the biology of sugar molecules, led to the development of tools for studying cell-cell interactions in immunology and oncology.
- π¬ The foundational bioorthogonal reaction discussed is the Staudinger Ligation, which was adapted from a classic reaction to create stable products for imaging in biological systems.
- π The use of zebrafish as a model organism for optical imaging was highlighted, demonstrating the ability to visualize glycans during development and cell division.
- π‘ The concept of 'click chemistry' was introduced, which involves the use of azides and alkynes in copper-catalyzed reactions, known for its speed and efficiency in forming triazoles.
- π The development of copper-free click chemistry was underscored as a significant advancement, enabling faster bioorthogonal reactions suitable for in vivo imaging.
- π οΈ The speaker's lab focused on the discovery of new bioorthogonal reactions, considering the vast potential of chemical space beyond the known biological reactivity.
- π The application of bioorthogonal chemistry in the biopharma industry was discussed, particularly in the development of antibody-drug conjugates and targeted therapeutics.
- 𧬠The potential for machine learning to revolutionize chemistry by predicting outcomes in areas with large datasets, such as catalysis and pharmaceuticals, was acknowledged.
Q & A
What is bioorthogonal chemistry?
-Bioorthogonal chemistry refers to chemical reactions that neither interact with nor interfere with biological systems. These reactions involve chemical functional groups that do not exist in nature and can be used for various applications, including imaging and drug delivery.
Why is bioorthogonal chemistry considered a 'Berkeley story' according to the speaker?
-The speaker considers bioorthogonal chemistry a 'Berkeley story' because the foundational work in this field was conducted at Berkeley between 1996 and 2010 before the speaker moved to Stanford in 2015.
What was the initial focus of the speaker's research during their PhD and postdoctoral training?
-During their PhD and postdoctoral training, the speaker's focus was on organic synthesis and how it could be applied to study biological processes, particularly in the field of glycoscience, which involves the biology of sugar molecules or glycans.
How did the speaker's interest in studying processes in biological systems influence their research direction?
-The speaker's interest in studying processes in biological systems led them to explore the idea of performing chemical reactions in less controllable biological settings, such as living cells, laboratory animals, and potentially humans, for purposes like attaching imaging probes to molecules of interest.
What is the significance of azides in bioorthogonal chemistry?
-Azides are significant in bioorthogonal chemistry because they are small, chemically reactive groups that do not exist in nature and are inert in biological systems. They can be used to introduce chemically reactive groups into biomolecules for imaging and other applications.
What was the first bioorthogonal reaction developed by the speaker's lab?
-The first bioorthogonal reaction developed by the speaker's lab was a modification of the classic Staudinger reduction, known as the Staudinger ligation. This reaction involves the use of azides and triphenylphosphine, forming a stable product in biological settings.
How did the discovery of bioorthogonal reactions impact the study of glycans?
-The discovery of bioorthogonal reactions allowed researchers to label and image glycans in living systems, facilitating the study of changes in glycosylation patterns associated with diseases like cancer. This enabled non-invasive imaging techniques and advanced the understanding of glycans' roles in biological processes.
What challenges did the speaker's lab face with the Staudinger ligation, and how were they addressed?
-The main challenge with the Staudinger ligation was its slow reaction rate, which limited its effectiveness in living systems. This was addressed by developing faster bioorthogonal reactions, such as the copper-free click chemistry, which uses strained alkynes to accelerate the reaction without toxic catalysts.
What is the significance of the tetrazine ligation in bioorthogonal chemistry?
-The tetrazine ligation is significant because it is a very fast bioorthogonal reaction that uses an inverse-demand Diels-Alder reaction between trans-cyclooctene and tetrazine. This reaction is orders of magnitude faster than previous methods and can be used for applications like pro-drugging strategies and in vivo chemical reactions.
How has bioorthogonal chemistry been applied in the biopharma industry?
-Bioorthogonal chemistry has been applied in the biopharma industry for making antibody-drug conjugates (ADCs) with site-specific drug attachment, enhancing targeted therapeutic capabilities. Companies have developed platform technologies using these chemistries to create more effective and precise cancer treatments and other therapeutic applications.
Outlines
π Academic Rivalry and Bioorthogonal Chemistry Origins
The speaker playfully addresses the academic rivalry between Stanford and Berkeley, expressing disappointment at the lack of enthusiasm from the Stanford attendees. They introduce the topic of Bioorthogonal Chemistry, a field that has its roots in Berkeley between 1996 and 2010. The speaker's research at Berkeley focused on the basic science aspect, while their work at Stanford has been more translational. The backstory of Bioorthogonal Chemistry is tied to the speaker's PhD and postdoctoral training, with a focus on glycoscienceβthe study of sugar molecules and their role in cell interactions and diseases. The speaker reminisces about their time as a graduate student in organic synthesis and the foundational tool of organic chemists, the round bottom flask, which allows for precise control over chemical reactions. This control is essential for the chemist to perform reactions that have been developed over a century. The speaker's interest in conducting chemistry in less controllable biological settings, such as living cells or animals, led to the concept of Bioorthogonal Chemistry.
𧬠The Concept of Bio-orthogonal Chemistry
The speaker delves into the concept of Bio-orthogonal Chemistry, which involves chemical reactions that do not interfere with biological systems. They describe the universe of all possible chemical reactions and identify a 'galaxy' within it known as the biological reactivity space, which includes all the chemicals found in the human body. The speaker's lab aimed to discover a bio-orthogonal reactivity space, which would consist of chemical functional groups not found in nature and would not crosstalk with biological chemicals. This idea was driven by the speaker's interest in glycoscience and the challenges of imaging sugars in living systems. The speaker also discusses their experience as a postdoctoral fellow at UCSF, studying changes in cell surface glycans associated with diseases like cancer, and the lack of technology for imaging sugars at the time.
π¬ The Advent of Bioorthogonal Chemistry
The speaker recounts the development of Bioorthogonal Chemistry, beginning with their idea for imaging sugars in living systems during their postdoctoral years. They describe attending a conference where they learned about the biosynthesis of cylic acid and the potential for chemically modified metabolic precursors to be incorporated into cell surface glycans. Inspired by this, the speaker proposed a two-step process for imaging sugars in living systems, which involved feeding cells a chemically modified simple sugar and then using a complementary chemical group to react with the sugar and form a bond with an imaging probe. This approach required the development of bio-orthogonal chemical groups that would selectively react with each other without interfering with biological processes. The speaker admits they did not know what these functional groups could be at the time, but this idea became the foundation for their future research.
π§ͺ The Staudinger Ligation: A Foundational Bioorthogonal Reaction
The speaker introduces the Staudinger Ligation as the first bioorthogonal reaction developed in their lab. This reaction is a modification of the classic Staudinger reduction, which involves the reaction between triphenylphosphine and an azide to form an intermediate called an Aza illid. The speaker explains how they adapted this reaction to create a stable product by introducing a methyl ester on one of the benzene rings, allowing the intermediate to cyclize and form an amide bond, resulting in a stable cyclic amide. This stable product enabled the reaction to be used for imaging experiments in living systems, such as attaching imaging probes to sugars. The speaker also discusses the process of incorporating azides into various sugars and using the Staudinger Ligation to conjugate them with phosphine reagents for imaging purposes in living cells and animals.
π Advancing Bioorthogonal Chemistry for Faster Reactions
The speaker discusses the limitations of the Staudinger Ligation in terms of reaction kinetics, which was too slow for many imaging applications. They needed a reaction that was at least two orders of magnitude faster. Around the early 2000s, there was an interest in the reactivity of azides, particularly their ability to act as one-three dipoles in dipolar cyclo additions with alkynes, a chemistry known as click chemistry. However, the classic Huisgen cyclo addition was too slow and required heating, which is not viable for living systems. The speaker's lab was exploring ways to accelerate the azide-alkyne cyclo addition without using a toxic metal catalyst. They were inspired by the concept of ring strain in organic chemistry and considered the reactivity of strained alkynes with azides, which led to the discovery of a faster bio-orthogonal reaction.
π The Discovery of Copper-Free Click Chemistry
The speaker describes the discovery of a copper-free click chemistry, which was a significant advancement in bioorthogonal chemistry. This new reaction was based on the use of ring-strained alkynes and azides, which was inspired by the teachings of organic chemistry regarding the reactivity of strained rings. The speaker's team found that a cyclooctyne with a phenylazide reacted explosively, indicating a highly reactive and fast reaction. This discovery was a promising alternative to the copper-catalyzed click chemistry, which was not suitable for biological systems due to the toxicity of copper. The team's work on this copper-free click chemistry opened up new possibilities for fast and efficient bioorthogonal reactions in living systems.
π Bioorthogonal Chemistry in Zebrafish Development
The speaker shares how the developed bioorthogonal reactions were applied to study glycosylation changes in zebrafish, a model organism for vertebrate development. The zebrafish's translucent body allows for easy optical imaging, and its rapid development from a single cell to a larval fish in just five days provides a unique opportunity to observe the entire developmental process. The speaker's team used metabolic and chemical labeling techniques to integrate azidosugars into cell surface glycans during development. By adding a bioorthogonal reactive group, such as difo linked to a fluorescent dye, they could label and image the sugars incorporated into cell surface glycans at different time points. This approach led to the discovery of the role of sugars in cell division and tissue development.
Mindmap
Keywords
π‘Bioorthogonal chemistry
π‘Glycoscience
π‘Staudinger ligation
π‘Click chemistry
π‘Copper-free click chemistry
π‘Zebrafish
π‘Antibody-drug conjugates (ADCs)
π‘Machine learning
π‘Glycan
π‘Hyaluronic acid
π‘Tetrazine ligation
Highlights
Introduction of the concept of bio-orthogonal chemistry, which allows chemical reactions to occur without interfering with biological systems.
Development of the Staudinger Ligation, a foundational bio-orthogonal reaction for imaging sugars in living systems.
The need for faster bio-orthogonal reactions for in vivo imaging, leading to the development of copper-free click chemistry.
Utilization of strained alkynes to accelerate azide-alkyne cycloaddition without toxic metal catalysts.
Discovery of the HIPS ligation, an adaptation of the Pictet-Spengler reaction for site-specific antibody-drug conjugates.
Application of bio-orthogonal chemistry in the biopharma industry for targeted therapeutics like site-specific antibody drug conjugates (ADCs).
Invention of the Aldehyde Tag technology for the site-specific attachment of drugs to antibodies.
Introduction and application of the Tetrazine Ligation, a fast bio-orthogonal reaction used in human clinical trials.
Use of bio-orthogonal chemistry for the development of pro-drugs that activate only in specific microenvironments.
The potential of bio-orthogonal chemistry to improve spatiotemporal control in drug delivery.
Opportunities for new bio-orthogonal reactions discovery, emphasizing the vast area of unexplored chemical space.
The impact of machine learning on chemistry, particularly in reaction development and catalysis.
Challenges and future prospects in reading and writing glycans, with advances in mass spectrometry and potential for DNA sequencing-like approaches.
The intersection of academic research with startup culture and the drive to translate scientific discoveries into real-world impact.
Cultural shift towards entrepreneurship among students and the integration of startup goals into academic research projects.
Personal reflection on the transition from curiosity-driven research to a focus on impactful applications and entrepreneurship.
Transcripts
Browse More Related Video

Nobel Prize lecture: Carolyn Bertozzi, Nobel Prize in Chemistry 2022

The Map of Chemistry
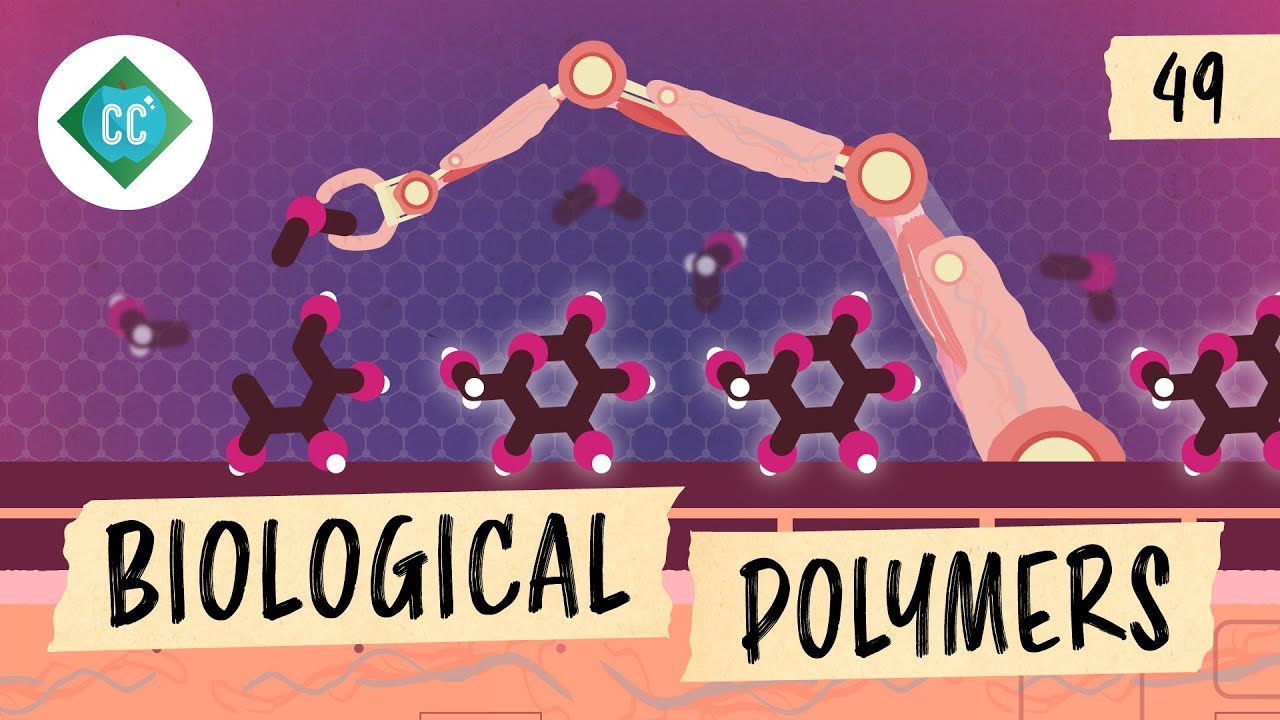
Biological Polymers: Crash Course Organic Chemistry #49
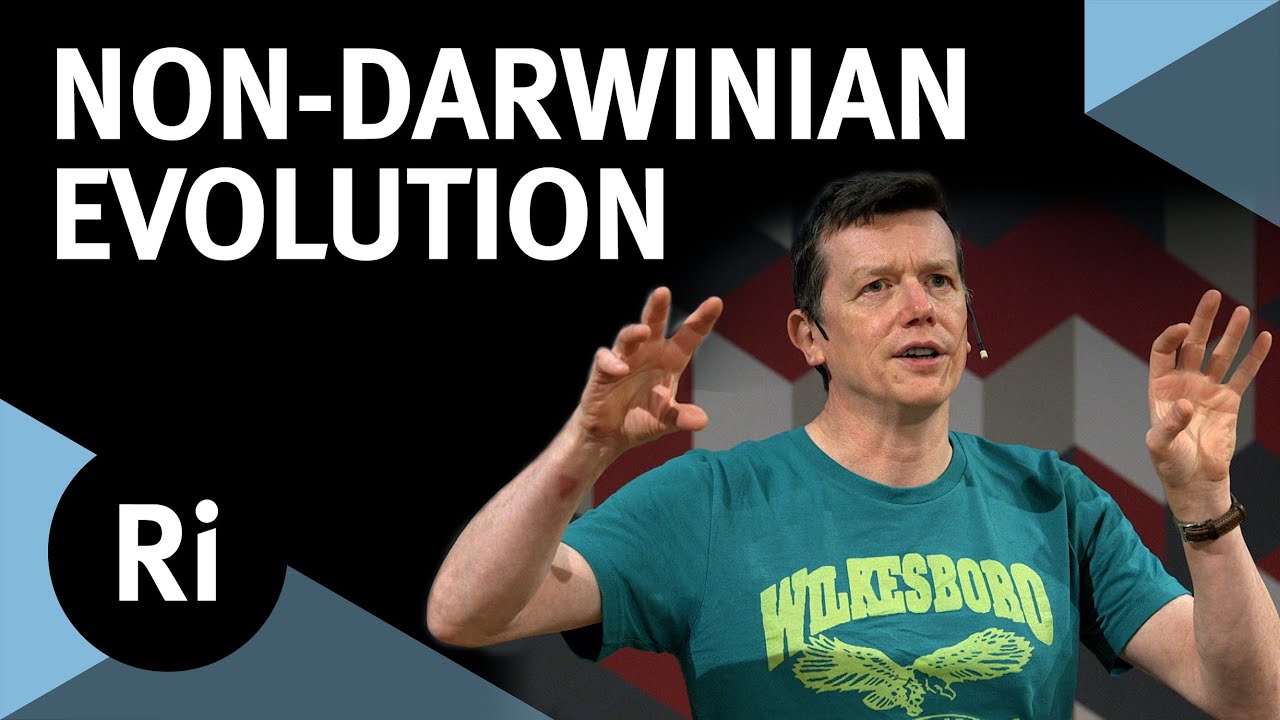
What Darwin won't tell you about evolution - with Jonathan Pettitt
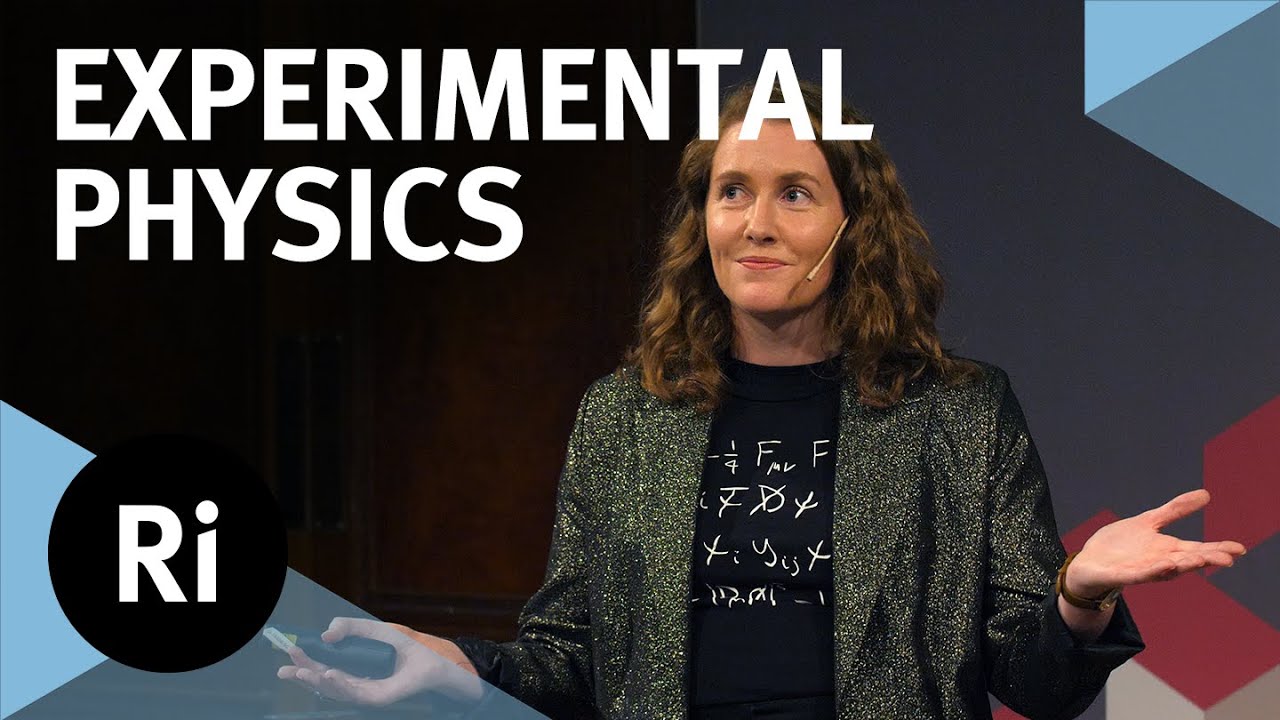
Physics experiments that changed the world β with Suzie Sheehy
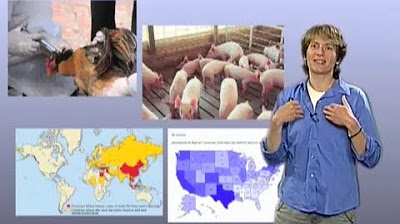
Carolyn Bertozzi (UC Berkeley) Part 1: Chemical Glycobiology
5.0 / 5 (0 votes)
Thanks for rating: