27. Nuclear Materials β Radiation Damage and Effects in Matter
TLDRThe video script is a detailed lecture on the effects of radiation on nuclear materials, as part of an MIT OpenCourseWare series. The professor delves into the multi-scale nature of radiation damage, starting from atomic defects and moving up to the structural changes seen in nuclear reactors. The lecture covers material science basics, explaining the properties of materials and how radiation can alter them. It introduces concepts such as displacement, thermal spikes, and the formation of voids and interstitial clusters due to radiation. The professor also discusses the importance of understanding an undamaged structure to assess radiation damage. The lecture includes an interactive segment where the audience predicts the outcomes of compressing various materials using a hydraulic press, highlighting the differences in material properties like ductility, stiffness, strength, toughness, and hardness. The goal is to understand how radiation impacts these properties, which is crucial for the safe operation and maintenance of nuclear reactors.
Takeaways
- π The lecture provides an introduction to nuclear materials and their behavior under radiation, emphasizing the importance of understanding the interaction between radiation and materials at various scales, from atomic to engineering scales.
- β’οΈ Radiation can cause significant damage to materials, leading to defects that can accumulate and alter the material's properties, which is crucial for applications like nuclear reactors where radiation damage is a primary concern.
- π΅οΈββοΈ The process of radiation damage begins with the displacement of atoms from their lattice sites, creating vacancies and interstitials, which can then move and interact, leading to the formation of clusters and eventually voids or interstitial clusters.
- π¬ Voids, or pockets of vacuum in materials, can cause swelling and dimensional changes, which are problematic in nuclear reactors as they can impede the flow of coolant and lead to a loss of reactor cooling capacity.
- π§ Material science concepts are fundamental to understanding radiation effects, including the structure of crystalline solids, point defects like vacancies and interstitials, and how they influence a material's properties.
- π High-quality imaging techniques like Transmission Electron Microscopy (TEM) allow for the direct observation of atomic defects, providing evidence for the theoretical concepts discussed in material science.
- π οΈ Dislocations, line defects in the crystal lattice, are key to plastic deformation in materials. They can move through slip and climb mechanisms, which can be influenced by radiation-induced defects.
- π Grain boundaries act as sinks for radiation-induced defects, making materials with smaller grains or nano-grains more resistant to radiation damage compared to those with larger grains.
- π The mechanical properties of materials, such as stiffness (Young's modulus), strength (yield or tensile strength), toughness (energy to fracture), ductility (extent of deformation before fracture), and hardness (resistance to deformation), are all distinct and can be affected by radiation.
- π© The behavior of materials under stress, such as in a hydraulic press, can be surprising and is determined by their combination of mechanical properties. For example, ductility does not necessarily equate to toughness.
- π Even the hardest known natural material, diamond, can shatter under compressive stress due to its low toughness, demonstrating that hardness and toughness are independent properties.
Q & A
What is the significance of understanding nuclear materials and radiation damage?
-Understanding nuclear materials and radiation damage is crucial because it involves knowing everything from the atomic level to the engineering scale. This knowledge helps in comprehending how radiation interacts with matter, causes defects, and leads to material failure in nuclear reactors.
What is a thermal spike in the context of radiation damage?
-A thermal spike is a very small, localized zone of melting that occurs when an incoming particle like a neutron displaces an atom, transferring a significant amount of energy and causing a group of atoms to reach extremely high temperatures for a very short period, less than a picosecond.
How do voids form in materials due to radiation damage?
-Void formation begins with individual atoms, known as vacancies, that are displaced from their original positions by radiation. These vacancies can cluster together, creating a localized region of lower density or even a pocket of vacuum within the material, known as a void.
What is the role of dislocations in material deformation?
-Dislocations are defects in the crystal lattice that allow for plastic deformation without breaking the material. They can move through a process called slip, where they slide through the lattice by breaking and reforming a line of bonds, which requires less energy than breaking an entire plane of bonds.
Why are grain boundaries significant in the context of radiation damage?
-Grain boundaries are significant because they can act as sinks or destroyers of radiation damage. They can accommodate many point defects without being destroyed, making materials with smaller grains or nano-grains more resistant to radiation damage.
What is the difference between a vacancy and an interstitial in a crystal lattice?
-A vacancy is a point defect where an atom is missing from its regular position in the lattice, while an interstitial is an atom that occupies an irregular position, typically in the space between normal lattice sites. Vacancies are easier to create but harder to move, whereas interstitials are harder to create but easier to move.
How does the presence of inclusions affect a material's properties?
-Inclusions are 3-D defects that consist of a piece of a different material within a pure material. They can disrupt the regular lattice structure and act as stress concentrators, potentially making the material more susceptible to fracture or altering its mechanical properties.
What is the relationship between the size of a material's grains and its resistance to radiation damage?
-Materials with smaller grains or nano-grains are generally more resistant to radiation damage because grain boundaries can act as sinks for radiation-induced defects, reducing their accumulation and the consequent damage.
What is the difference between a material being ductile and being brittle?
-A ductile material can undergo significant plastic deformation before failure, whereas a brittle material tends to fracture with little or no plastic deformation. Ductility is associated with the movement of dislocations, while brittleness is associated with fracture when atomic bonds break.
How does work hardening affect the mechanical properties of a material?
-Work hardening is a process where cold working or plastic deformation of a material increases its strength due to the accumulation of dislocations. This makes it more difficult for dislocations to move, increasing the material's hardness and yield strength but reducing its ductility.
What is the role of dislocations in the context of radiation damage?
-Dislocations can interact with radiation-induced defects such as vacancies and interstitials. They can move and multiply under stress, leading to the formation of dislocation loops, which can cause the material to deform plastically. However, if dislocations accumulate or are blocked by defects, they can lead to increased stress and potential fracture.
Outlines
π Introduction to Nuclear Materials and Radiation Damage
The paragraph introduces the topic of nuclear materials and radiation damage, emphasizing the importance of understanding radiation's interaction with matter. It outlines the multi-scale nature of radiation damage, from atomic defects to large-scale reactor issues. The speaker, a professor, plans to cover material science basics, radiation's role in altering material properties, and the various ways materials can fail due to radiation damage. The concept of displacement damage, where incoming particles displace atoms from their lattice sites, is introduced. The paragraph also touches on thermal spikes and the resulting defects that accumulate to form radiation damage.
π¬ Understanding Void Formation and Crystalline Structures
This section delves into the formation of voids in materials, their shape, and how they are influenced by crystalline structures. Voids, which are pockets of vacuum in materials, are shown to often align in a specific direction, contrary to expectations of a random distribution. The size of these voids is discussed, with mention of how they can cause dimensional changes in materials like fuel rods in reactors. The importance of understanding undamaged structures to assess radiation damage is highlighted, followed by a brief introduction to crystalline solids, their regular lattice arrangements, and the impact of these arrangements on physical properties.
π Point Defects and Their Impact on Material Properties
The paragraph discusses point defects in a perfect crystal, such as vacancies and interstitials, which are zero-dimensional defects involving single atoms out of place. It explains how vacancies can be detected through quenching studies and how they increase with temperature. The movement and clustering of vacancies and interstitials are described, including the formation of split dumbbell interstitials. The energetics of point defects is also explored, highlighting the difficulty of creating interstitials and their ease of movement, in contrast to vacancies which are easier to create but harder to move.
π© Dislocations and Their Role in Material Deformation
This section focuses on dislocations, which are line defects in the crystal lattice that contribute to material deformation. The difference between edge and screw dislocations is explained, along with their movement mechanisms, glide (or slip), and climb. The impact of dislocation interactions, such as pile-up and the resulting increase in material strength through work hardening, is discussed. The importance of dislocations in material science and their influence on plasticity is emphasized.
π§ Material Response to Stress: Slip versus Fracture
The paragraph explores the balance between slip and fracture in materials when subjected to stress. It describes how materials prefer to deform through slip rather than fracture, and how dislocations facilitate this process. The role of dislocation types, such as screw and edge dislocations, in material response to stress is highlighted. The concept of work hardening is introduced, explaining how the accumulation of dislocations can increase a material's strength during plastic deformation.
ποΈ Grain Boundaries and Their Interaction with Defects
This section discusses grain boundaries, which are two-dimensional defects that form when two crystals with different orientations meet. The ability of grain boundaries to act as sinks for radiation damage and their role in the resistance of nano-grain materials to radiation damage is explained. The concept of twins, another type of two-dimensional defect, is introduced. The paragraph also covers inclusions, which are three-dimensional defects, and their interaction with dislocations in influencing material deformation and fracture.
π Material Properties: Stiffness, Strength, Toughness, and More
The paragraph delves into various material properties, clarifying common misconceptions about terms like stiffness, strength, toughness, and ductility. It explains the concept of a stress-strain curve and how it relates to these properties. The importance of understanding these properties is emphasized, especially in the context of how radiation can alter them. The distinction between hardness and the other properties is also discussed, highlighting hardness as a measure of resistance to plastic deformation.
π¨ Observations of Material Deformation and Failure
This section presents a series of observations and experiments demonstrating the deformation and failure of different materials under stress. It includes the compression of an aluminum canister, the behavior of brass and copper coins, the deformation of a lead ball, and the surprising plasticity of a steel ball bearing. The discussion highlights how real-world observations of material behavior can differ from theoretical expectations, and the importance of considering multiple material properties when predicting outcomes.
π Diamonds and the Concept of Material Brittleness
The final paragraph focuses on diamonds, contrasting their extreme hardness with their lack of toughness. It describes an experiment where a diamond is compressed, leading to its shattering, and highlights the concept of brittleness in materials. The discussion emphasizes the importance of understanding the interplay between a material's hardness, ductility, and the energy required for fracture, as well as the role of defects in influencing these properties.
Mindmap
Keywords
π‘Radiation Damage
π‘Displacement
π‘Thermal Spike
π‘Interstitials and Vacancies
π‘Void Swelling
π‘Crystalline Solid
π‘Dislocations
π‘Grain Boundaries
π‘Stress-Strain Curve
π‘Material Properties
π‘Work Hardening
Highlights
The lecture focuses on the study of nuclear materials and their interaction with radiation, emphasizing the importance of understanding the behavior of materials in nuclear reactors.
The material science primer introduces fundamental concepts such as atomic defects, material properties, and the impact of radiation on these properties.
Radiation can cause various types of defects in materials, including vacancies, interstitials, voids, and dislocations, which can lead to material failure.
The professor explains the multi-scale nature of radiation damage, ranging from femtoseconds to gigaseCONDS and from angstroms to meters.
A thermal spike is a localized zone of melting caused by the displacement of atoms due to radiation, leading to an increase in temperature and potential material damage.
Void swelling is a phenomenon where materials expand due to the accumulation of radiation defects, which can affect the performance and safety of nuclear reactors.
The lecture discusses the different types of defects in materials, including point defects, dislocations, grain boundaries, twins, and inclusions, and their effects on material properties.
The movement of dislocations, which are defects in the crystal lattice, is crucial for the plastic deformation of materials and plays a significant role in radiation damage.
Grain boundaries act as sinks for radiation damage, making small-grain and nano-grain materials more resistant to radiation effects.
The professor provides a detailed explanation of material properties such as stiffness, strength, toughness, ductility, and hardness, and how they differ from one another.
A stress-strain curve is introduced as a graphical representation of a material's response to stress, highlighting its elastic and plastic deformation regions.
The lecture includes an interactive component where the audience is invited to predict the outcome of various materials being compressed or deformed, testing their understanding of material properties.
The professor clarifies the difference between ductile and brittle materials, emphasizing the role of dislocation movement and the energy required for deformation.
The lecture concludes with an examination of the stress-strain curves of familiar materials, reinforcing the concepts discussed throughout the session.
The importance of understanding material properties is emphasized in the context of nuclear engineering, as it directly impacts the safety and efficiency of nuclear reactors.
Transcripts
Browse More Related Video
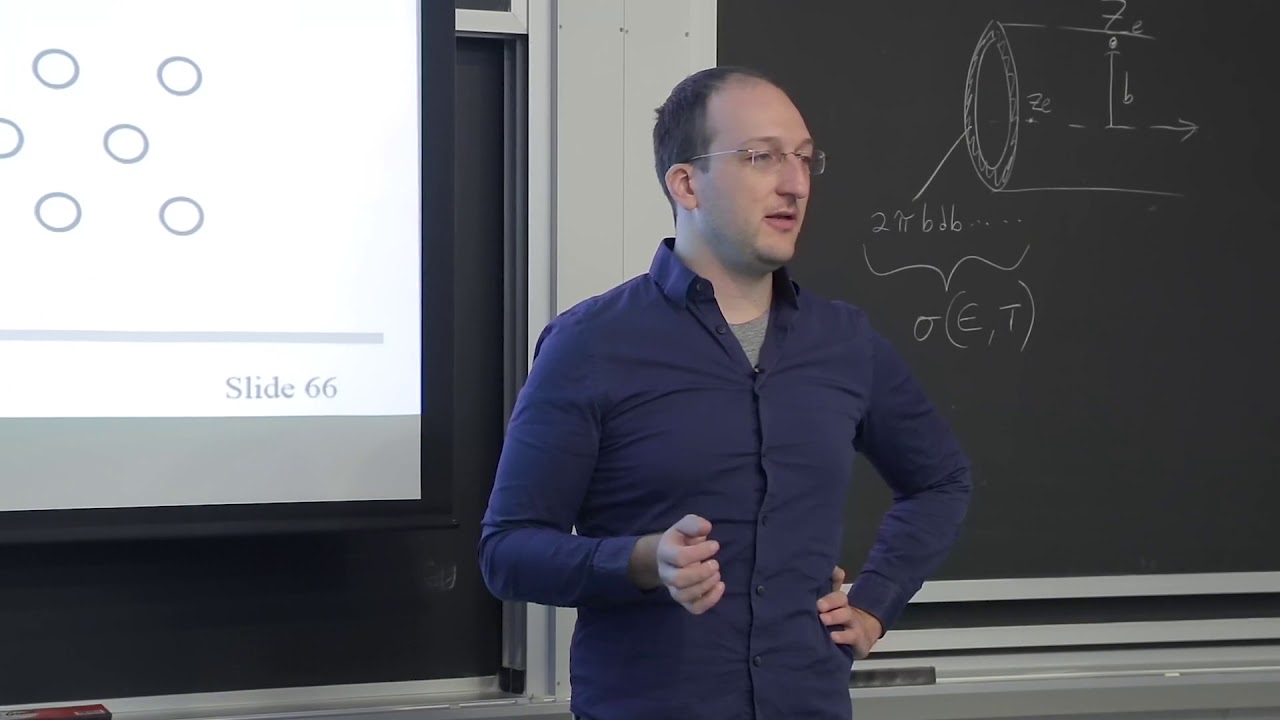
29. Nuclear Materials Science Continued
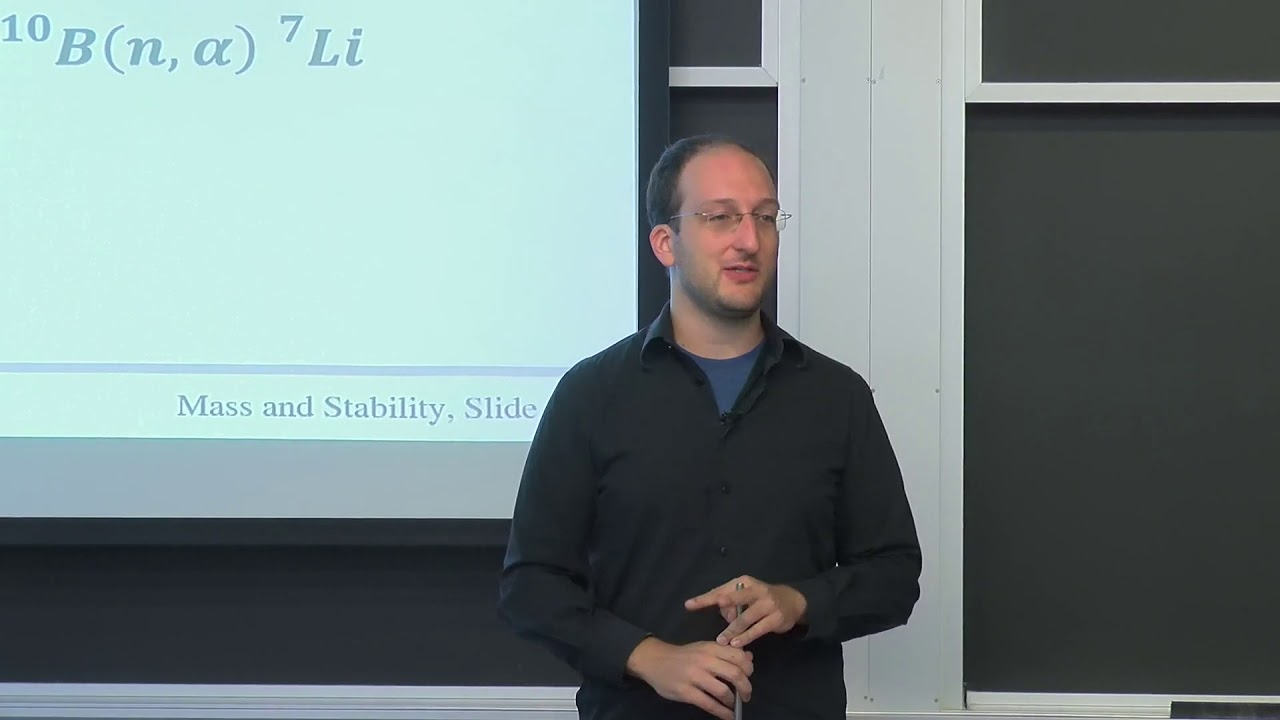
3. Nuclear Mass and Stability, Nuclear Reactions and Notation, Introduction to Cross Section

18. Ion-Nuclear Interactions II β Bremsstrahlung, X-Ray Spectra, Cross Sections
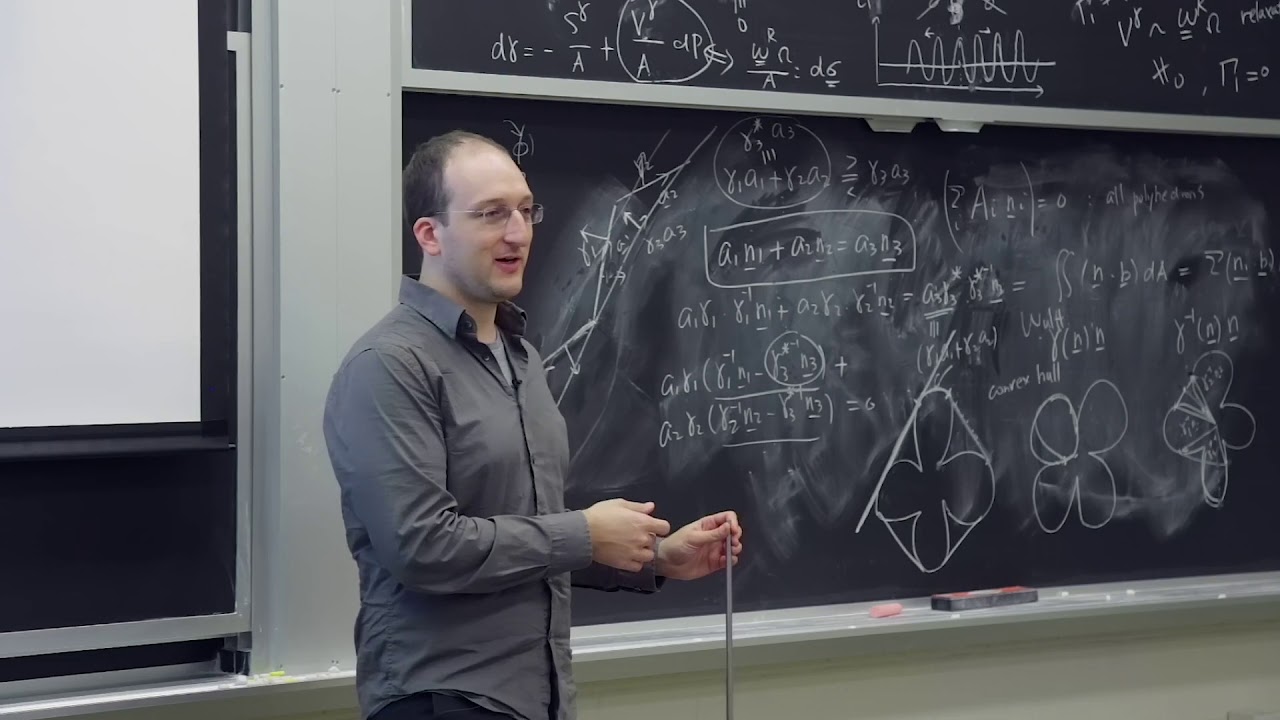
31. Frontiers in Nuclear Medicine, Where One Finds Ionizing Radiation (Background and Other Sources)
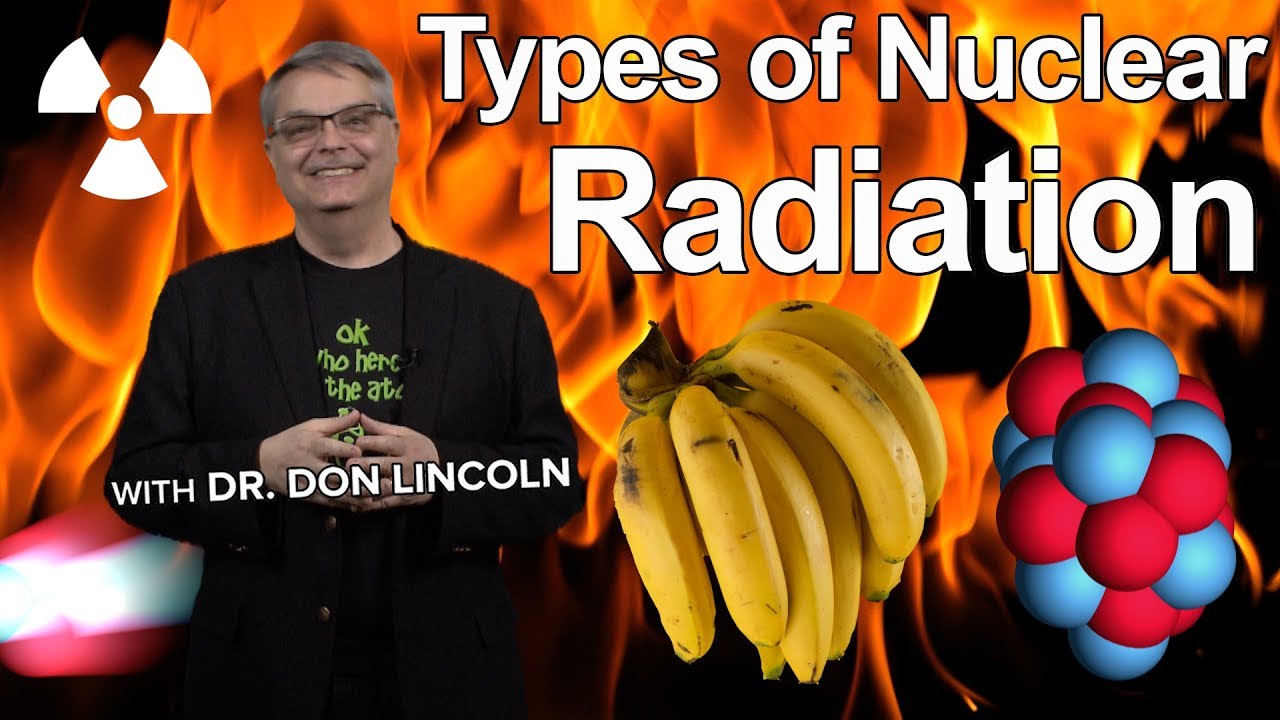
Types of Nuclear Radiation

33. Long-Term Biological Effects of Radiation, Statistics, Radiation Risk
5.0 / 5 (0 votes)
Thanks for rating: