Clarius: Fundamentals of Ultrasound 1 (Physics)
TLDRThis script delves into the fundamentals of ultrasound imaging, explaining how transducers emit sound waves that reflect off tissues, creating images. It covers the physics of ultrasound, including wave propagation, reflection, and attenuation, and discusses the importance of frequency in image clarity and penetration. The use of gel for acoustic coupling and the impact of various factors on image quality are highlighted, providing a comprehensive guide to ultrasound technology and its clinical applications.
Takeaways
- 🌐 Understanding the basic physics and clinical concepts of ultrasound imaging is crucial for effective use.
- 🛰️ Ultrasound imaging systems use a transducer, or probe, to send and receive sound waves within the patient's body.
- 🔊 Sound waves in ultrasound are mechanical pressure waves that propagate through the body and are reflected off different tissues.
- 📡 The transducer contains piezoelectric elements that generate pressure waves when excited by an electric current.
- 🖼️ Ultrasound images are cross-sections formed line by line in front of the transducer, showing organs in black and white.
- 🚀 Sound waves travel rapidly through human tissue at approximately 1540 meters per second.
- 🔍 The system interprets the signal from the returning echoes to display structures at their exact location on the screen.
- 🌗 Attenuation of sound waves occurs as they travel through tissue, with absorption converting acoustic energy into heat, making deeper structures harder to see.
- 🔄 Reflection happens when sound waves encounter a boundary between two different media, such as tissue and bone, creating an acoustic shadow.
- 🌫 Scatter occurs when ultrasound waves meet a non-homogeneous surface, resulting in speckle and a grainy appearance in the image.
- 💧 Acoustic coupling is necessary for sound to travel through the body, requiring the use of gel to ensure proper contact between the transducer and the patient's skin.
- 🔉 The frequency of sound waves affects the clarity of ultrasound images, with lower frequencies providing better penetration and higher frequencies offering better resolution for shallow structures.
Q & A
What is an ultrasound and how does it work?
-An ultrasound is a medical imaging technique that uses high-frequency sound waves to create images of the body's internal structures. It works by using a transducer, or probe, to send sound waves into the patient's body. These waves bounce off different tissues and return to the transducer as echoes, which are then processed to produce a visual image of the organs.
What are the components of an ultrasound transducer?
-An ultrasound transducer contains several piezoelectric elements that are excited by an electric current. This causes the elements to expand and contract, creating pressure waves that propagate through the body.
How is an ultrasound image formed?
-An ultrasound image is a cross-section inside the patient that is formed line by line in front of the transducer. As the user positions the transducer at different angles, the electric current applied to the elements generates a transmitted pulse or sound wave that propagates through the patient, creating the image.
What is the speed at which sound travels through human tissue during an ultrasound scan?
-Sound travels rapidly through human tissue at approximately 1540 meters per second during an ultrasound scan.
How does the ultrasound system interpret the returning sound waves to display structures on the screen?
-Since sound travels back and forth at a known speed, the system can interpret the signal and display structures at their exact location on the screen. Shallow structures that reflect first are displayed at the top of the image, while echoes from deeper structures, which take longer to return, are displayed at the bottom.
What is the major source of sound wave attenuation in soft tissue during an ultrasound scan?
-The major source of sound wave attenuation in soft tissue is absorption, which is the conversion of acoustic energy into heat, causing the ultrasound wave to become weaker over distance.
How do ultrasound systems compensate for weaker deep signals?
-Ultrasound systems compensate for weaker deep signals by increasing the time gain compensation (TGC) or gain at deeper levels, which brightens deeper structures to improve visibility.
What is reflection in the context of ultrasound imaging?
-Reflection occurs when a sound wave encounters a boundary between two different media, such as tissue and bone. Some of the wave bounces back toward the source, creating an echo. Structures like bone are strong reflectors, reflecting most of the incoming wave and often creating an acoustic shadow.
What is scatter and how does it affect ultrasound images?
-Scatter occurs when ultrasound waves encounter a medium with a non-homogeneous surface, causing a portion of the sound wave to be scattered in random directions. The consequence of scatter is known as speckle, which produces the grainy appearance in an ultrasound image.
Why is acoustic coupling important for ultrasound imaging?
-Acoustic coupling is important because ultrasound is reflected by air. To ensure proper contact between the transducer and the patient's skin, gel is used to avoid air trapping, which would reflect sound waves and create shadowing.
How do sound frequencies affect the clarity of an ultrasound image?
-Sound frequencies affect the clarity of an ultrasound image in that lower frequencies are less attenuated and provide better penetration for deeper structures, while higher frequencies offer better resolution for shallow structures. The selection of the transducer with the appropriate frequency is critical for optimal imaging of the specific anatomy being examined.
Outlines
🌐 Understanding Ultrasound Basics
This paragraph introduces the fundamental physics and clinical concepts behind ultrasound imaging. It explains how an ultrasound transducer, or probe, emits sound waves into the body, which then bounce off various tissues and return as echoes. These echoes are processed to create a visual representation of the internal organs. The paragraph delves into the mechanics of the ultrasound transducer, which contains piezoelectric elements that generate pressure waves when stimulated by electric current. It also discusses how the speed of sound in human tissue allows for the precise location of internal structures on the ultrasound image. Additionally, it touches on the concepts of sound wave attenuation, reflection, and the importance of acoustic coupling for effective imaging, as well as the impact of frequency on image quality and the visibility of deeper structures.
🔊 Sound Wave Characteristics in Ultrasound Imaging
The second paragraph focuses on the characteristics of sound waves in the context of ultrasound imaging. It defines the cycle and wavelength of a sound wave and explains how frequency is measured in Hertz. The audible range for humans is contrasted with the ultrasonic range used in medical imaging, which is much higher. The paragraph further discusses how the size of the piezoelectric elements in the transducer affects the frequency of the sound waves produced, and how this in turn influences the image clarity and the ability to penetrate deeper tissues. It highlights the trade-off between resolution and penetration depth, and the importance of selecting the appropriate transducer frequency for the specific anatomy being examined. The summary also mentions the challenges of imaging behind solid structures or air due to absorption and reflection, and the role of ultrasound systems in amplifying weaker signals for better visibility.
Mindmap
Keywords
💡Ultrasound
💡Transducer
💡Piezoelectric Element
💡Echo
💡Attenuation
💡Time Gain Compensation (TGC)
💡Reflection
💡Acoustic Shadow
💡Scatter
💡Speckle
💡Frequency
💡Acoustic Coupling
Highlights
Ultrasound imaging systems use a transducer to send sound waves into the patient's body, which bounce off different tissues and return as echoes to create images.
The ultrasound transducer contains piezoelectric elements that expand and contract to generate pressure waves for imaging.
An ultrasound image is a cross-section formed line by line in front of the transducer, showing organs at different depths.
Sound waves travel through human tissue at approximately 1540 meters per second, allowing for real-time imaging.
Ultrasound systems interpret the speed of sound to display structures at their exact locations on the screen.
Attenuation of sound waves is primarily due to absorption, which weakens the wave over distance, affecting visibility of deeper structures.
Time gain compensation (TGC) is used to brighten deeper structures and improve their visibility in ultrasound images.
Reflection occurs when sound waves encounter boundaries between different media, creating echoes that form images.
Acoustic shadows are created by strong reflectors like bone, which reflect most of the incoming wave.
Scatter happens when ultrasound waves meet non-homogeneous surfaces, leading to speckle and a grainy image appearance.
Acoustic coupling is necessary for ultrasound waves to travel through the body, typically achieved with gel.
Sound frequencies are crucial for ultrasound imaging, with lower frequencies providing better penetration and higher frequencies offering better resolution.
The frequency range for typical ultrasound imaging is between 1 and 20 megahertz, affecting image clarity and depth.
Selecting the right transducer frequency is essential for optimal imaging of different anatomical structures.
Absorption may prevent imaging behind solid structures due to the conversion of acoustic energy into heat.
Reflection may hinder imaging behind solid structures or air due to the bounce-back of sound waves.
Transcripts
Browse More Related Video
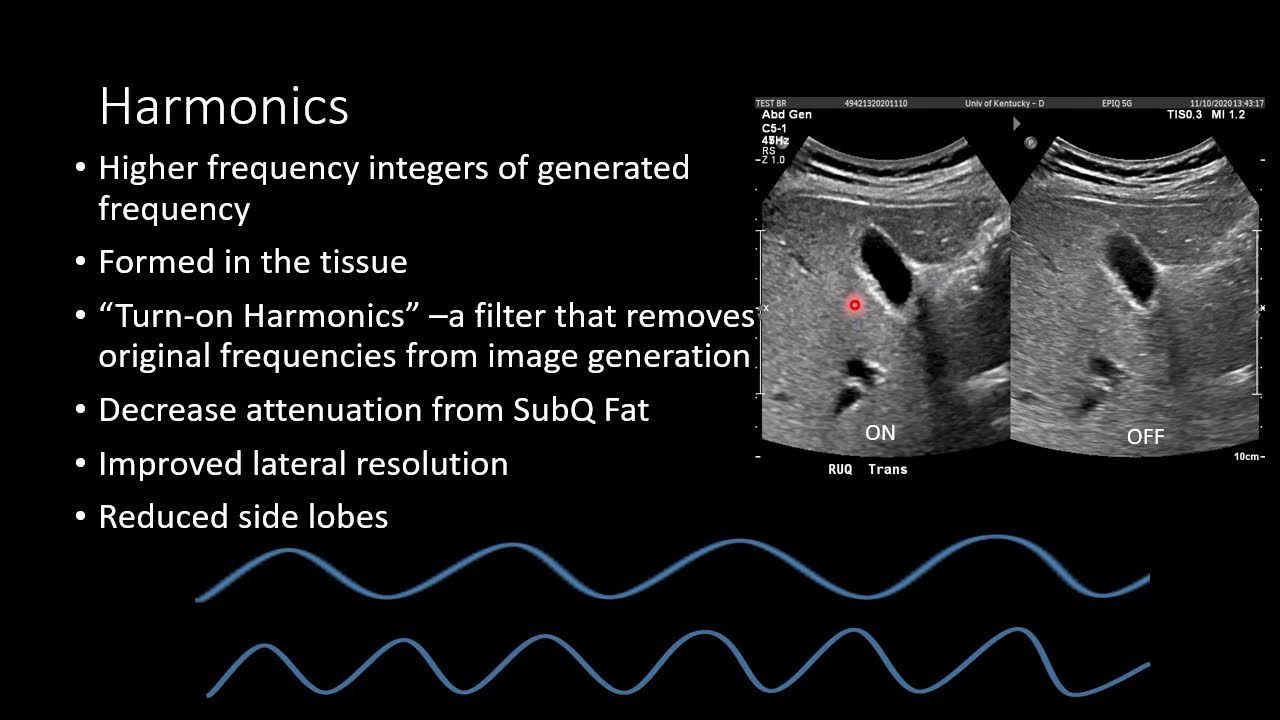
Ultrasound Physics - Image Generation
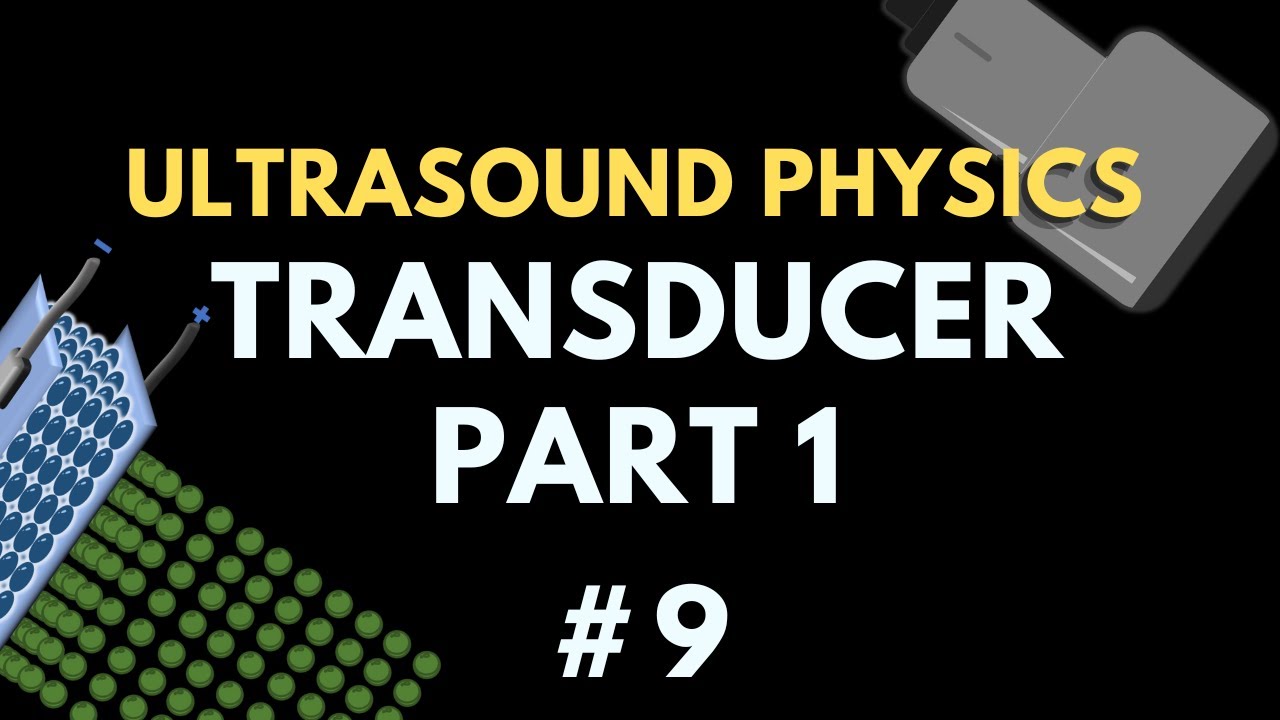
Ultrasound Transducer (Part 1) Piezoelectric Material and Matching Layer | Ultrasound Physics #9
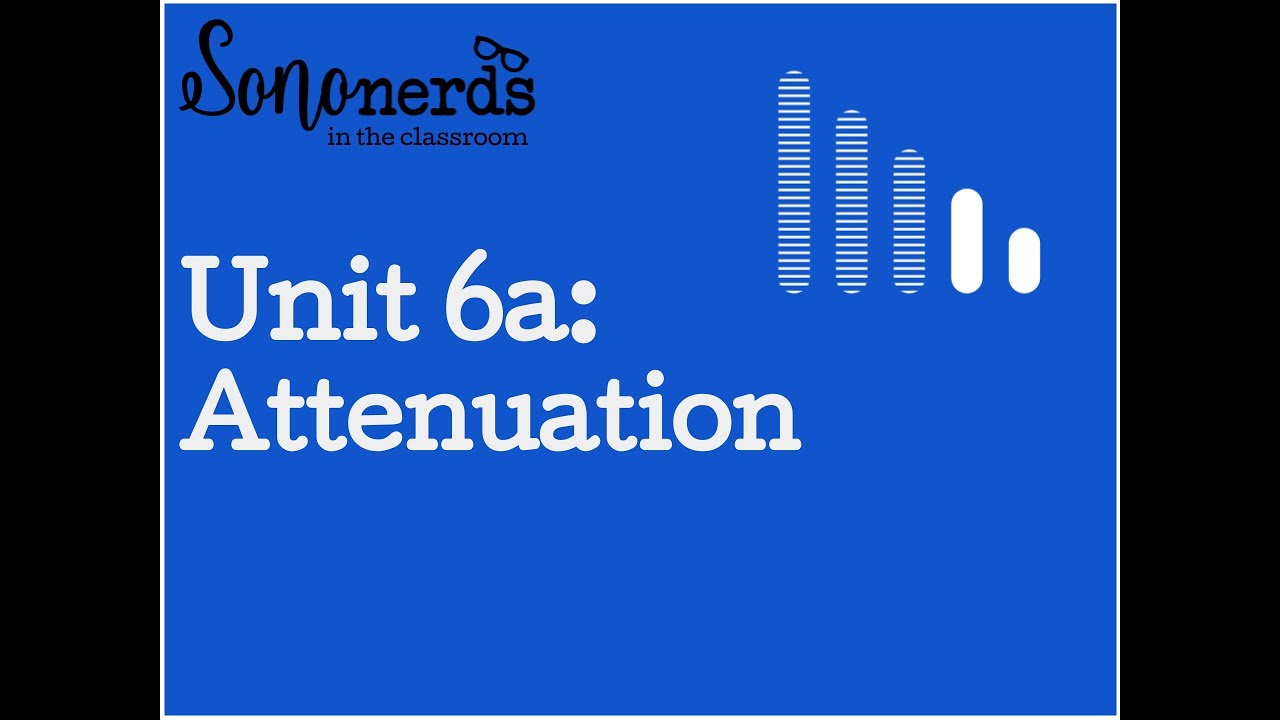
Ultrasound Physics with Sononerds Unit 6a
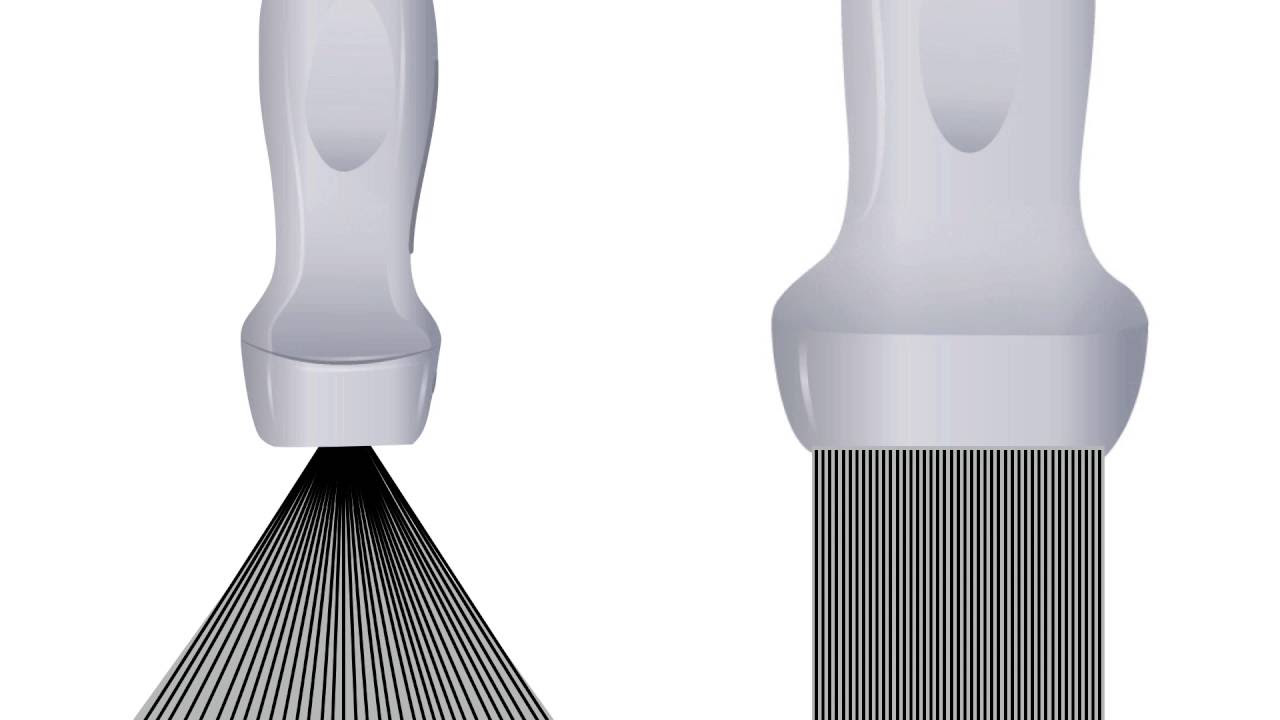
Basic Ultrasound Physics for EM
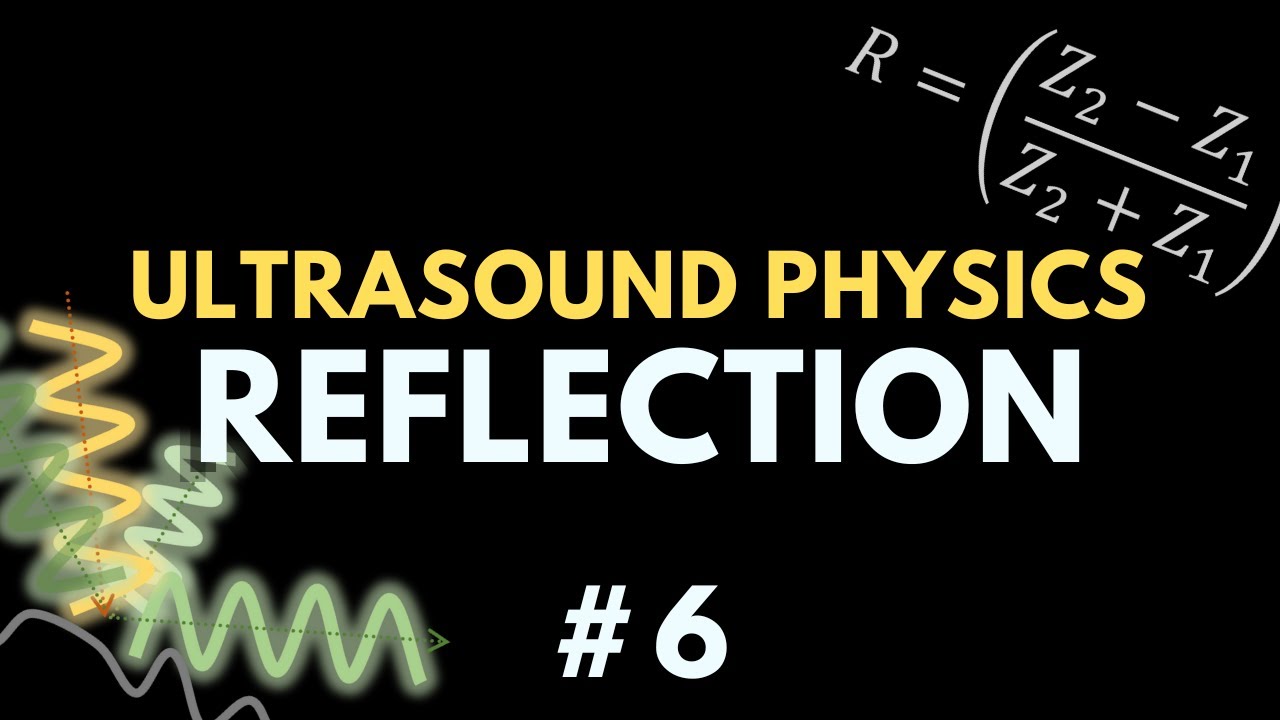
Reflection, Ultrasound Interaction with Matter | Ultrasound Physics | Radiology Physics Course #6
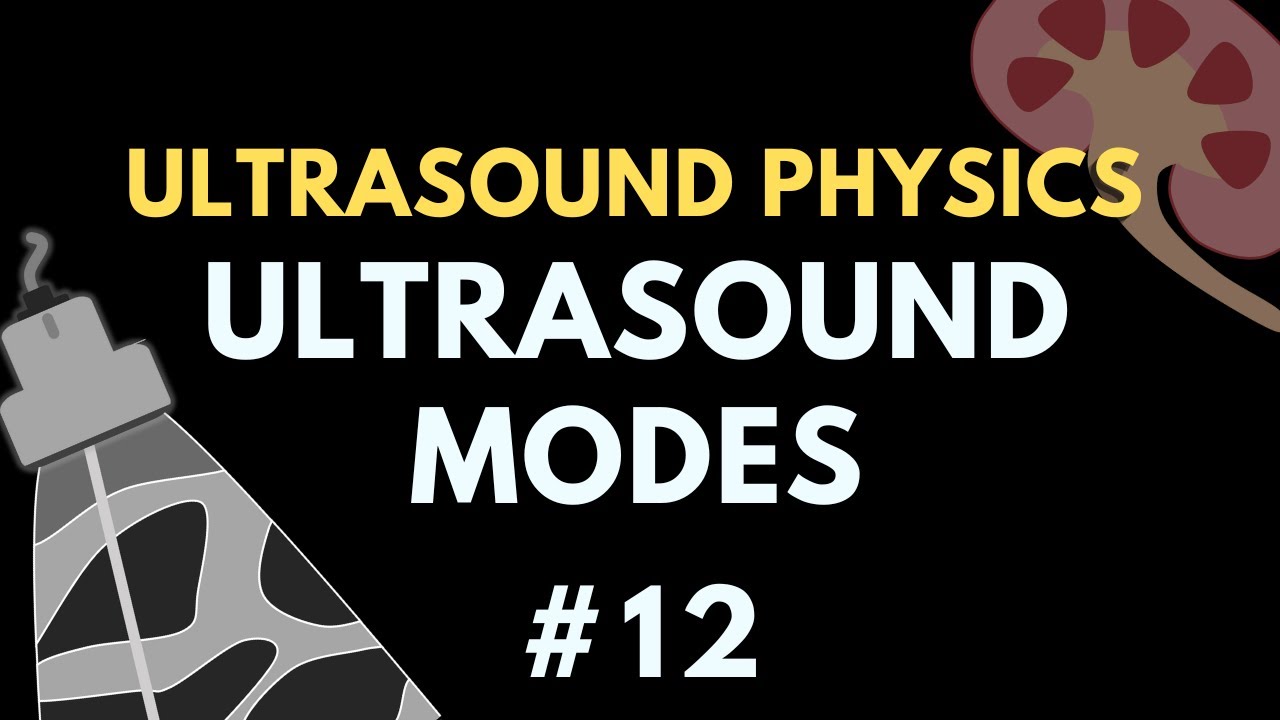
Ultrasound Modes, A, B and M Mode| Ultrasound Physics | Radiology Physics Course #12
5.0 / 5 (0 votes)
Thanks for rating: