Ultrasound Modes, A, B and M Mode| Ultrasound Physics | Radiology Physics Course #12
TLDRThis script delves into the fundamentals of ultrasound imaging, focusing on the generation and interpretation of ultrasound pulses. It explains how piezoelectric materials create and receive ultrasound waves, and the significance of acoustic impedance in reflecting these waves. The script explores different ultrasound modes, particularly A-mode for detecting tissue boundaries, B-mode for creating grayscale images of tissue structures, and M-mode for visualizing motion over time. It also touches on the importance of time gain compensation to adjust for signal attenuation as ultrasound waves travel through tissue.
Takeaways
- π Ultrasound pulses are generated and received by the piezoelectric material in the transducer.
- π The electronic signal from the returning echoes is interpreted using different ultrasound modes, including A, B, and M modes.
- π A-mode (Amplitude mode) detects tissue boundaries with varying acoustic impedances by measuring the amplitude of the returning echoes.
- π B-mode (Brightness mode) creates a two-dimensional image by scanning multiple lines and converting the amplitude of echoes into grayscale values.
- π» M-mode (Motion mode) combines A and B modes to display the movement of structures over time in a single scan line.
- β± The pulse repetition frequency and period affect the depth and resolution of B-mode images.
- π Ultrasound waves attenuate as they travel through tissue, affecting the intensity and amplitude of the returning echoes.
- π Time gain compensation is used to correct for the attenuation of ultrasound waves at different depths.
- π¬ Scattered waves, not just reflected waves from tissue boundaries, contribute to the echo texture seen in B-mode imaging.
- ποΈ The human eye perceives a continuous image in B-mode when the scan lines are updated at least 24 times per second.
- π A-mode is rarely used today but has specific applications in ophthalmology and detecting midline shifts in neonates.
Q & A
What is the role of piezoelectric material in ultrasound imaging?
-Piezoelectric material in ultrasound imaging is responsible for both creating and receiving ultrasound pulses. It converts electrical signals into mechanical vibrations (ultrasound waves) and vice versa, capturing the returning echoes.
How does the A-mode ultrasound scanning work?
-A-mode, or Amplitude mode, involves taking a single line of ultrasound data into the tissues. It detects tissue boundaries with differing acoustic impedance values and represents the amplitude of the returning echoes, which correspond to the tissue boundaries.
Why is the speed of sound in soft tissue important in ultrasound imaging?
-The speed of sound in soft tissue is crucial for calculating the distance or depth of tissue boundaries. It helps in determining the time it takes for the ultrasound wave to reach a boundary and return, which is then used to calculate the depth using the formula: depth = (time * speed of sound) / 2.
What is the significance of acoustic impedance in ultrasound imaging?
-Acoustic impedance is significant because it affects how much of the incident wave is reflected back towards the ultrasound machine and how much is transmitted through. Differences in acoustic impedance at tissue boundaries determine the strength of the reflected echoes.
How does the B-mode ultrasound scanning differ from A-mode?
-B-mode, or brightness mode, scans multiple lines across the tissue, similar to A-mode, but it then converts these amplitudes into a grayscale value to create a 2D image that represents the tissue boundaries and echo texture of the scanned area.
What is the minimum frame rate required for the human eye to perceive a continuous image in B-mode ultrasound?
-For the human eye to perceive a continuous image in B-mode ultrasound, the scanning process needs to happen at least 24 times within one second, which is equivalent to a frame rate of 24 frames per second.
What is the purpose of the M-mode or motion mode in ultrasound imaging?
-M-mode, or motion mode, combines elements of A-mode and B-mode to assess the movement of structures over time. It plots the A-mode signals as a brightness mode over time, allowing for the visualization of motion within the axial plane, such as the movement of the heart walls.
Why is time gain compensation used in ultrasound imaging?
-Time gain compensation is used to correct for the attenuation of the ultrasound beam as it travels through tissue. It ensures that echoes returning from deeper tissues are amplified to compensate for the loss in intensity, providing a more accurate representation of the tissue.
How does the frequency of an ultrasound wave affect its attenuation?
-The higher the frequency of an ultrasound wave, the faster it is attenuated as it travels through tissue. This is because higher frequency waves have shorter wavelengths, which are more easily scattered and absorbed by tissues.
What is the typical speed of sound used in soft tissue for ultrasound calculations?
-The average speed of sound used in soft tissue for ultrasound calculations is 1514 meters per second.
What are some specific uses of A-mode ultrasound imaging?
-A-mode ultrasound imaging is specifically used in ophthalmology to determine the positions of the eye's lens, vitreous humor, and retina, and to identify any masses within the retina. Historically, it was also used to detect midline shifts in neonatal brains.
Outlines
π Ultrasound Generation and Interpretation
This paragraph introduces the fundamental concepts of ultrasound technology, focusing on how ultrasound pulses are generated and received by the transducer using piezoelectric materials. It explains the process of interpreting electronic signals from the returning echoes, and delves into the different modes of ultrasound scanning, specifically A, B, and M modes. The A-mode, which stands for amplitude, is highlighted as the simplest form of ultrasound imaging, relying on the differences in acoustic impedances at tissue boundaries to create a visual representation of these boundaries. The paragraph also discusses the attenuation of the ultrasound wave as it travels through tissue and how this affects the received echoes.
π Understanding A-Mode and B-Mode Ultrasound Imaging
The second paragraph delves deeper into the A-mode and B-mode ultrasound imaging techniques. It explains how A-mode captures a single line of information by firing a single transducer element, translating the amplitude of the returning echoes into a grayscale value that represents tissue boundaries. The B-mode, or brightness mode, is then introduced as an extension of A-mode, where multiple lines are scanned to create a two-dimensional image. The paragraph also discusses the importance of pulse repetition frequency and the need for rapid scanning to create a live, continuous image that the human eye can perceive. Additionally, it touches on the use of grayscale values to represent the strength of echoes and the concept of echo texture, which is influenced by the scattering of ultrasound waves within the tissue.
π Exploring M-Mode and Echo Texture in Ultrasound
The third paragraph explores the M-mode, or motion mode, which combines elements of A-mode and B-mode to track the movement of tissues over time. It describes how an A-mode scan line can be superimposed on a B-mode image to observe changes in tissue, such as the contraction of the heart's ventricles. The paragraph also discusses the importance of echo texture in providing a detailed view of the tissue being imaged, which is influenced by the scattering of ultrasound waves. The M-mode is highlighted as a valuable tool for assessing motion within the axial plane, despite the advent of Doppler mode, which measures blood flow velocity.
π οΈ Time Gain Compensation in Ultrasound Imaging
The final paragraph addresses the issue of ultrasound beam attenuation as it travels through tissue, which can affect the accuracy of echo interpretation. It introduces the concept of time gain compensation, a technique used to adjust for the loss of signal intensity at greater depths. The paragraph explains that as the ultrasound wave travels deeper into the tissue, its intensity decreases exponentially, which can lead to misinterpretation of the echo signals. Time gain compensation is presented as a solution to amplify the returning echoes from deeper tissues, ensuring a more accurate representation of the tissue structure and function.
Mindmap
Keywords
π‘Ultrasound
π‘Piezoelectric material
π‘Echoes
π‘Acoustic impedance
π‘A-mode
π‘B-mode
π‘M-mode
π‘Doppler scanning
π‘Pulse repetition frequency
π‘Time gain compensation
Highlights
Ultrasound pulses are generated and received by the piezoelectric material in the transducer.
The electronic signal from received echoes is interpreted using different ultrasound modes.
A-mode ultrasound uses amplitude to detect tissue boundaries with varying acoustic impedances.
In A-mode, the absence of echo indicates similar acoustic impedance between tissues.
A-mode can be used for specific applications like in Ophthalmology to determine the eye's internal structures.
B-mode, or brightness mode, creates images by scanning multiple lines and converting amplitudes to grayscale values.
B-mode images require at least 24 scans per second for the human eye to perceive a continuous image.
M-mode, or motion mode, combines A-mode and B-mode to assess the movement of tissue over time.
M-mode is particularly useful for examining the heart's contractions and the movement of valves.
The speed of sound in soft tissue is used as an average for calculating ultrasound speed.
Pulse repetition frequency affects the depth and resolution of B-mode images.
Time gain compensation is necessary to correct for the attenuation of ultrasound waves as they travel through tissue.
The intensity of the ultrasound beam changes as it travels deeper into tissues, affecting image quality.
Scattered ultrasound waves contribute to the echo texture seen in B-mode images.
Doppler scanning, which measures blood flow, is reserved for a future section and is not covered in this talk.
A-mode's simplicity makes it less common in modern ultrasound imaging, with specific uses remaining.
B-mode is the most familiar ultrasound imaging mode, providing detailed images of tissue boundaries.
M-mode's relevance has decreased with the advent of Doppler mode but still offers value in daily practice.
Transcripts
Browse More Related Video
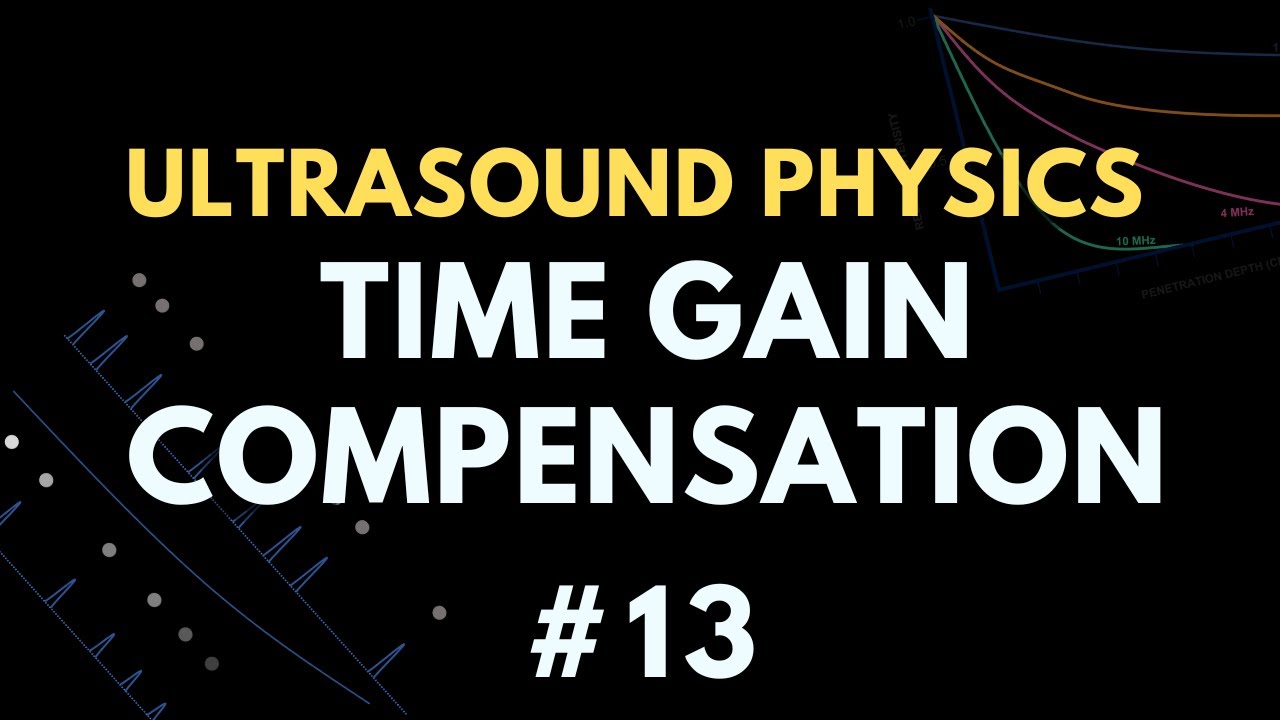
Time Gain Compensation | Ultrasound Physics | Radiology Physics Course #13
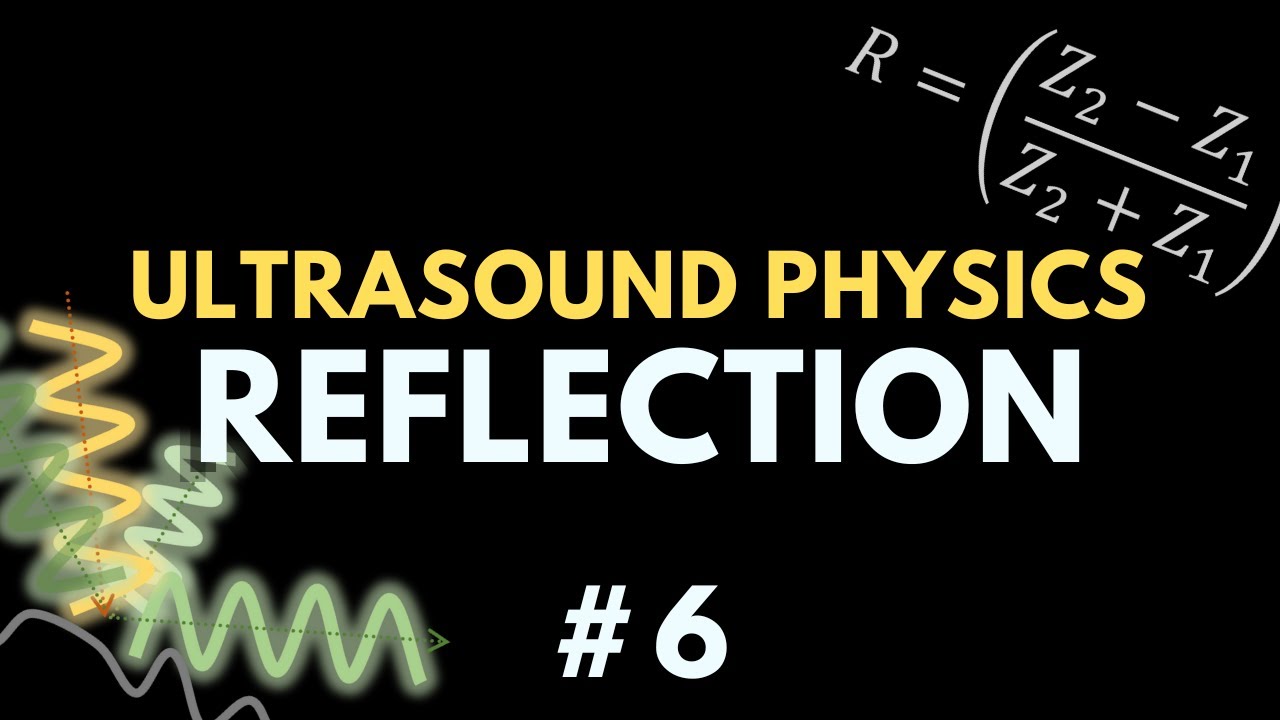
Reflection, Ultrasound Interaction with Matter | Ultrasound Physics | Radiology Physics Course #6
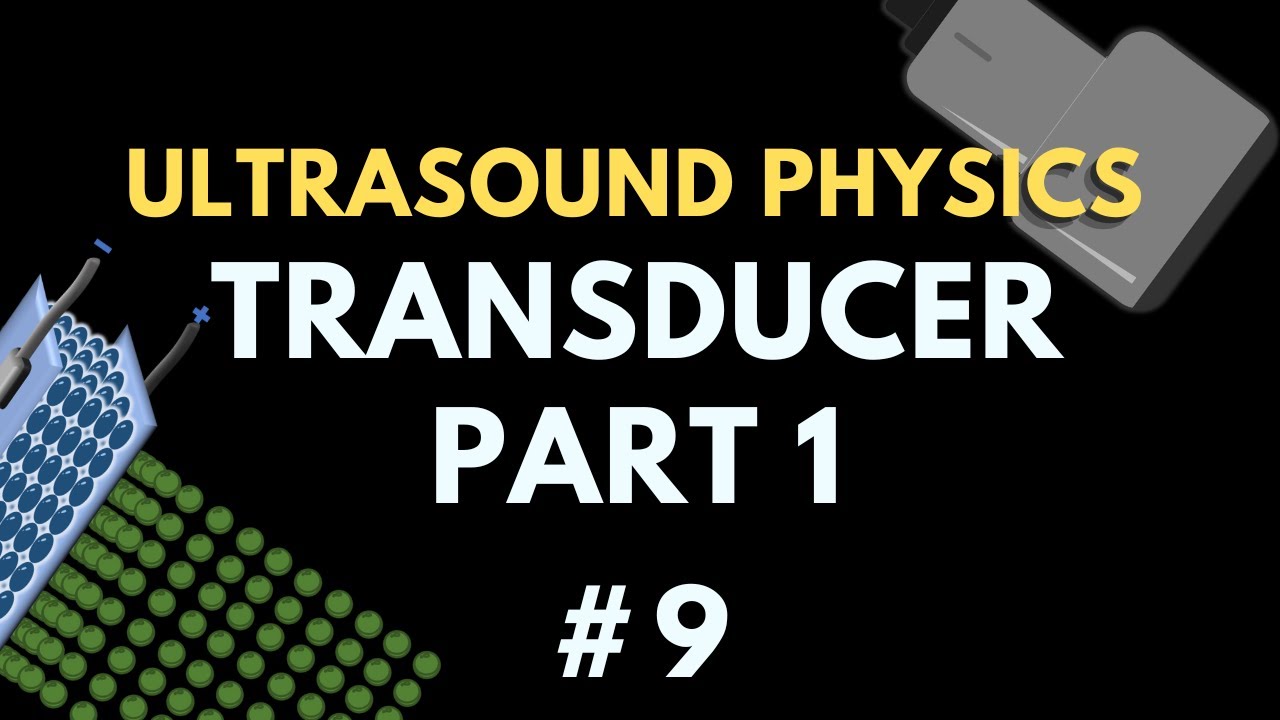
Ultrasound Transducer (Part 1) Piezoelectric Material and Matching Layer | Ultrasound Physics #9
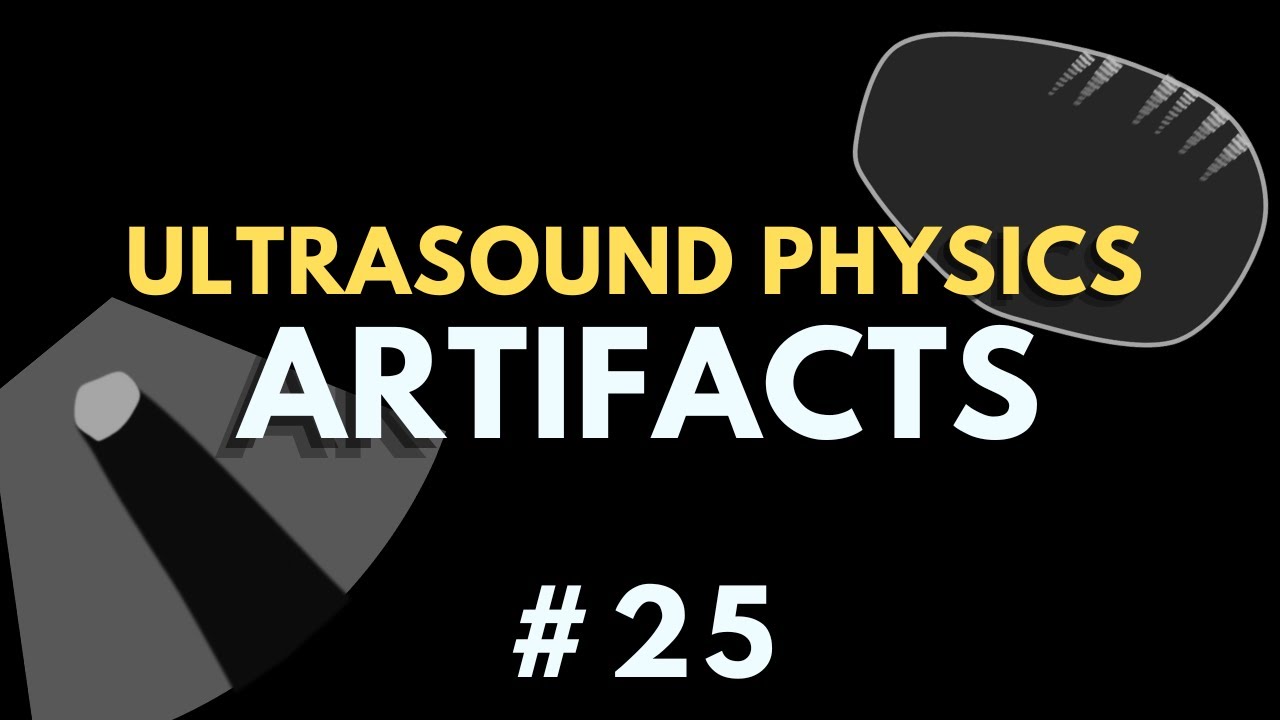
Ultrasound Artifacts | Ultrasound Physics Course | Radiology Physics Course #25

Ultrasound Scatter and Attenuation | Ultrasound Physics | Radiology Physics Course #8
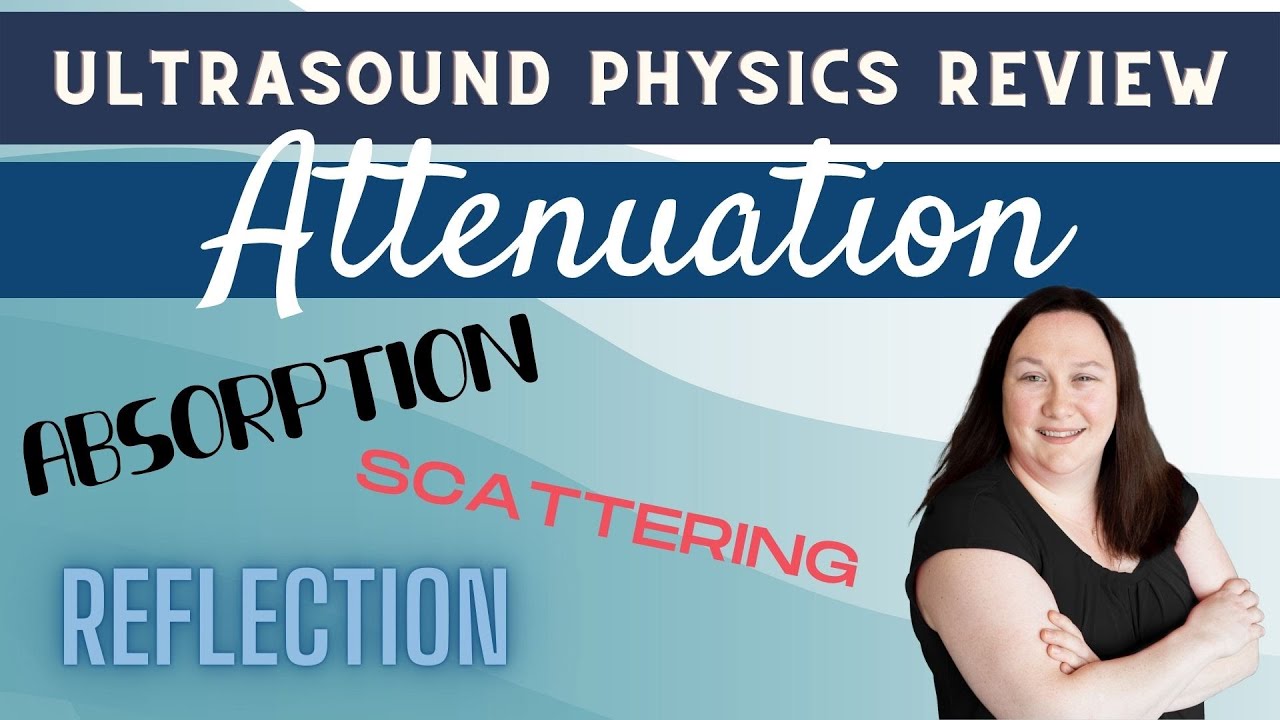
Ultrasound Physics Review | Attenuation | Sonography Minutes
5.0 / 5 (0 votes)
Thanks for rating: