Tara Shears - Antimatter: Why the anti-world matters
TLDRThe transcript discusses the enigmatic nature of antimatter, its importance in understanding the universe's evolution, and the current scientific efforts to explore it. It highlights experiments like AMS on the International Space Station, which detects antimatter in cosmic rays, and LHC's LHCb experiment, studying matter-antimatter differences at a quark level. The Alpha experiment at CERN aims to trap anti-hydrogen atoms for detailed analysis. Despite advancements, theθ° of why the universe is dominated by matter over antimatter remains, pointing to undiscovered physics and the potential role of neutrinos or supersymmetry.
Takeaways
- π Antimatter is not just science fiction but a crucial element for understanding the evolution of the universe.
- π¬ The behavior of antimatter is fundamental to our comprehension of particle physics and the early universe's conditions.
- π€ Despite its importance, our understanding of antimatter is limited and we know very little about its nature.
- π§ͺ Experiments are being conducted to bridge the knowledge gap, with three main approaches being utilized today.
- π The AMS experiment on the International Space Station is searching for antimatter in cosmic rays to understand its prevalence in the universe.
- π§² CERN's Large Hadron Collider (LHC) and LHCb experiment are studying antimatter by colliding protons and analyzing the resulting fundamental particles.
- π₯ The Alpha experiment at CERN is focused on creating and measuring antihydrogen atoms to compare with hydrogen atoms.
- π Current experimental results show a slight difference in the behavior of matter and antimatter, particularly under the weak force.
- π The existence of more antimatter in the universe than previously thought suggests unknown mechanisms of production.
- π€ The search for antimatter differences is an ongoing quest that may involve new physics beyond the standard model, such as supersymmetry.
- π Future advancements in antimatter research will likely come from continued analysis of existing data and the development of new experimental techniques.
Q & A
What is antimatter and why is it significant in understanding the universe?
-Antimatter is a type of matter composed of antiparticles, which have the same mass as particles of regular matter but have opposite charge and other particle properties. It is significant in understanding the universe because the behavior of antimatter could help us comprehend how the universe evolved. The difference between matter and antimatter under the weak force is a key aspect of the standard model of particle physics, and any deviation from the expected behavior could point to new physics or a deeper understanding of the universe's evolution.
How does the Large Hadron Collider (LHC) contribute to antimatter research?
-The Large Hadron Collider (LHC) contributes to antimatter research by providing an environment where high-energy collisions can create both matter and antimatter fundamental particles. These collisions recreate the high temperatures of the early universe, allowing scientists to study the properties of antimatter directly and compare them to matter to look for differences that could explain the universe's matter-antimatter asymmetry.
What is the significance of the B quark in antimatter research?
-The B quark is significant in antimatter research because matter and antimatter versions of the B quark exhibit some of the largest differences in behavior. This makes it an excellent candidate for studying the effects of matter and antimatter differences, as any discrepancies in the behavior of B quarks and their antimatter counterparts could provide insights into the fundamental nature of antimatter and the universe's evolution.
How does the Alpha experiment at CERN differ from other antimatter research experiments?
-The Alpha experiment at CERN differs from other antimatter research experiments in that it focuses on creating and trapping antihydrogen atoms to make precise measurements of their properties. Unlike other experiments that measure antimatter as it decays, Alpha aims to hold anti-atoms in place long enough to study them in detail, including testing gravity's effect on antimatter and comparing the structure of antihydrogen to regular hydrogen.
What is the current understanding of the matter-antimatter asymmetry in the universe?
-The current understanding of the matter-antimatter asymmetry in the universe, based on the standard model of particle physics, is that there should be a roughly equal amount of matter and antimatter. However, observations indicate that the universe is dominated by matter, with very little antimatter present. This discrepancy suggests that there is a small, yet significant, difference between the behavior of matter and antimatter that allowed matter to dominate after the Big Bang. The exact nature of this difference remains one of the biggest questions in physics.
What is the role of the Alpha Magnetic Spectrometer (AMS) in antimatter research?
-The Alpha Magnetic Spectrometer (AMS) plays a crucial role in antimatter research by detecting and analyzing cosmic rays in orbit around the Earth. AMS looks for anomalies in the ratio of positrons to electrons as a function of energy, which could indicate the presence of dark matter or other new physics contributing to the production of antimatter in the universe.
How does the LHCb experiment contribute to the study of matter-antimatter differences?
-The LHCb experiment contributes to the study of matter-antimatter differences by focusing on the properties of B quarks and their decays. By comparing the behavior of B quarks and their antimatter counterparts, LHCb aims to measure the differences directly and provide insights into the fundamental interactions that govern the asymmetry between matter and antimatter.
What are the implications of finding more antimatter in the universe than previously thought?
-Finding more antimatter in the universe than previously thought could have profound implications for our understanding of the cosmos. It might suggest the presence of unknown astrophysical sources of antimatter or even new physics beyond the standard model. This could lead to a reevaluation of our theories about the early universe and the processes that shaped its evolution.
What is the current experimental evidence for the existence of antimatter?
-The current experimental evidence for the existence of antimatter comes from various sources, including the discovery of the positron (the antimatter counterpart of the electron) in cosmic rays by Carl Anderson in 1932, and the production of antimatter particles in particle accelerators like the LHC. Additionally, experiments like AMS are designed to detect and study antimatter in cosmic rays, providing further evidence of its existence.
What is the role of neutrinos in the study of antimatter and the universe's evolution?
-Neutrinos are elusive subatomic particles that are difficult to detect and about which we know relatively little. There is a possibility that neutrinos or their antimatter counterparts (antineutrinos) could exhibit a greater difference in behavior between matter and antimatter than other particles, such as quarks. Next-generation neutrino experiments aim to explore this further and could potentially provide new insights into the nature of antimatter and the universe's evolution.
What is supersymmetry and how could it relate to antimatter research?
-Supersymmetry is a theoretical extension of the standard model of particle physics that predicts the existence of a new set of particles, known as superpartners, for each known particle. While supersymmetry does not have a specific explanation for matter-antimatter differences, it could potentially incorporate them and is one of several candidate theories that could explain these differences. Additionally, the lightest of these superpartners is a leading candidate for dark matter, which could be related to the observed excess of antimatter in the universe.
Outlines
π The Elusive Nature of Antimatter
Antimatter, a term often associated with science fiction, is a scientific reality with profound implications for our understanding of the universe. This paragraph delves into the fundamental role of antimatter in the evolution of the cosmos, highlighting our limited knowledge and the experimental efforts to unravel its mysteries. It introduces the concept of particle physics and sets the stage for a discourse on antimatter's significance and the ways we study it.
π¬ The Fundamental Forces and the Standard Model
This section provides an overview of the fundamental forces that govern the behavior of matter in the universe, including the weak force, electromagnetic force, strong force, and gravity. It also introduces the Higgs field, which imparts mass to fundamental particles. The paragraph emphasizes the elegance and success of the Standard Model of particle physics, which describes the universe's fundamental components and their interactions, but notes that it does not fully explain the nature of antimatter.
π€ The Discovery and Mystery of Antimatter
The discovery of antimatter is recounted, from its theoretical prediction by Dirac's equation to its experimental confirmation through the observation of positrons. The paragraph highlights the historical significance of antimatter and the realization that every particle has a corresponding antimatter counterpart. It also touches on the implications for the early universe, where a slight imbalance between matter and antimatter led to the universe's current composition.
π The Search for Antimatter in the Universe
The quest to understand the distribution of antimatter in the universe is explored, with a focus on the AMS experiment aboard the International Space Station. The AMS is designed to detect and analyze cosmic rays, including antimatter, in orbit. The paragraph discusses the potential for discovering more antimatter than previously thought, challenging our current understanding and possibly pointing to new physics.
π₯ The Large Hadron Collider and Antimatter Research
This section discusses the Large Hadron Collider (LHC) at CERN and its role in studying antimatter. The LHC's high-energy collisions recreate conditions of the early universe, allowing scientists to produce and compare matter and antimatter particles. The LHCb experiment, in particular, is highlighted for its focus on the B quark, where matter-antimatter differences are most pronounced. The paragraph also mentions the potential for future discoveries and the ongoing quest to understand the nature of antimatter.
π Antihydrogen and the Quest for Understanding
The production and study of antihydrogen atoms at CERN's Antiproton Decelerator are discussed, with the ALPHA experiment's efforts to trap and measure antihydrogen atoms. The goal is to compare antihydrogen to hydrogen to seek differences that could shed light on the nature of antimatter. The paragraph emphasizes the challenges of trapping neutral antihydrogen and the preliminary tests conducted to observe how antimatter interacts with gravity.
π The Standard Model and the Search for New Physics
The paragraph summarizes the current understanding of antimatter within the framework of the Standard Model, noting the consistency of experimental measurements with the model's predictions. It also acknowledges the limitations of our knowledge, suggesting that the unaccounted antimatter in the universe may be explained by undiscovered particles or new physical processes. The potential of supersymmetry and future experiments to provide insights into antimatter is highlighted.
π The Future of Antimatter Research
The conclusion emphasizes the ongoing nature of antimatter research, with plans to analyze more data from the AMS and LHC experiments, as well as the potential for new insights from antihydrogen studies and neutrino experiments. The paragraph suggests that the future may hold significant discoveries in the realm of antimatter, possibly leading to a deeper understanding of the universe's evolution and the fundamental laws of physics.
Mindmap
Keywords
π‘Antimatter
π‘Particle Physics
π‘Standard Model
π‘Big Bang
π‘Cosmic Rays
π‘LHC (Large Hadron Collider)
π‘CP Violation
π‘Antihydrogen
π‘Supersymmetry
π‘Neutrinos
Highlights
Antimatter is not just science fiction but a crucial aspect of understanding the universe's evolution.
Antimatter could help us comprehend how the universe evolved, but its behavior remains largely a mystery.
The Large Hadron Collider (LHC) at CERN allows scientists to recreate conditions of the early universe to study antimatter.
The existence of antimatter was first confirmed through Carl Anderson's discovery of the positron in 1932.
Antimatter and matter annihilate each other, releasing enormous amounts of energy.
The universe's composition is a mystery, with a significant imbalance between matter and antimatter.
The LHC's LHCb experiment is designed to study the differences between matter and antimatter at a fundamental particle level.
The Alpha experiment at CERN successfully trapped antihydrogen atoms, allowing for direct comparison with hydrogen.
AMS, an experiment on the International Space Station, has found evidence of more antimatter in the universe than previously thought.
The standard model of particle physics, while successful, lacks a complete explanation for antimatter's behavior.
Supersymmetry, a popular theory, could potentially explain antimatter and dark matter, but experimental evidence is lacking.
The future of antimatter research involves analyzing more data from existing experiments and exploring new physics.
The search for understanding antimatter is one of the biggest questions in physics and is actively being pursued.
Antimatter research could lead to revolutionary insights into the nature of the universe and its fundamental laws.
The study of antimatter is driven by experiments, as there is currently no underlying theoretical explanation for its observed differences from matter.
The behavior of antimatter is crucial for understanding the universe's evolution from the Big Bang to its current state.
Transcripts
Browse More Related Video

Does Antimatter Create Anti-Gravity?
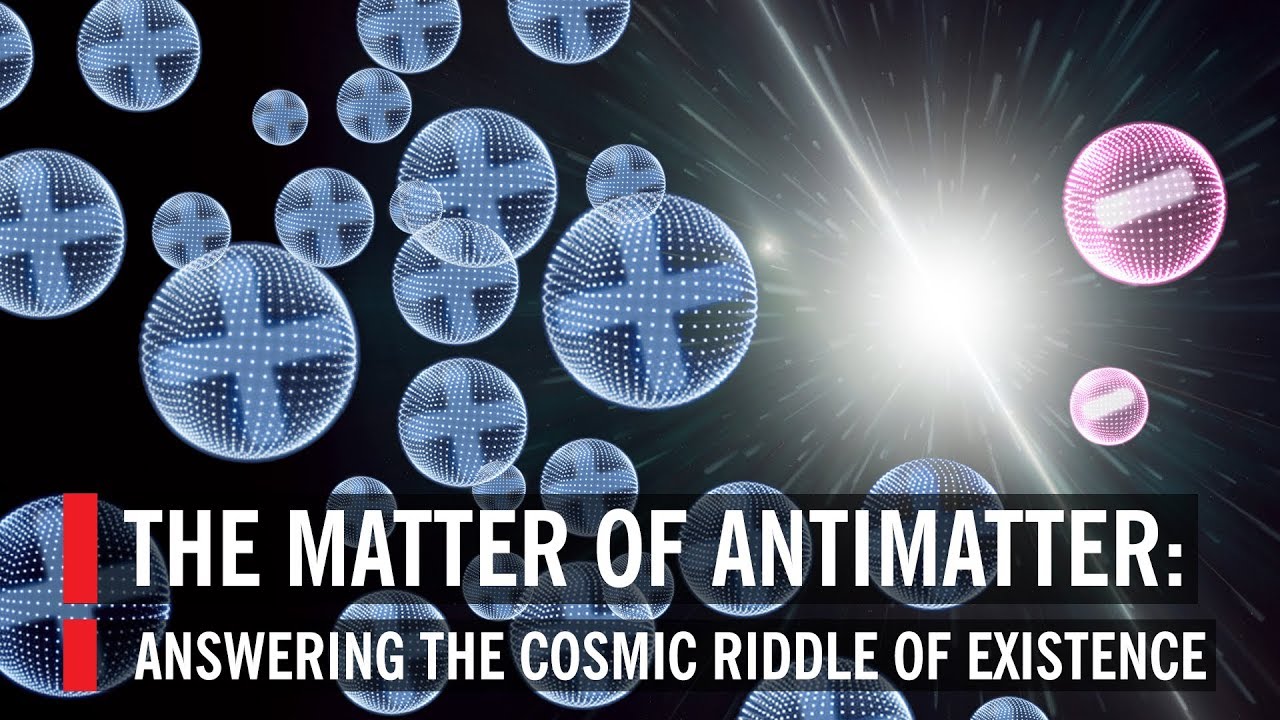
The Matter Of Antimatter: Answering The Cosmic Riddle Of Existence
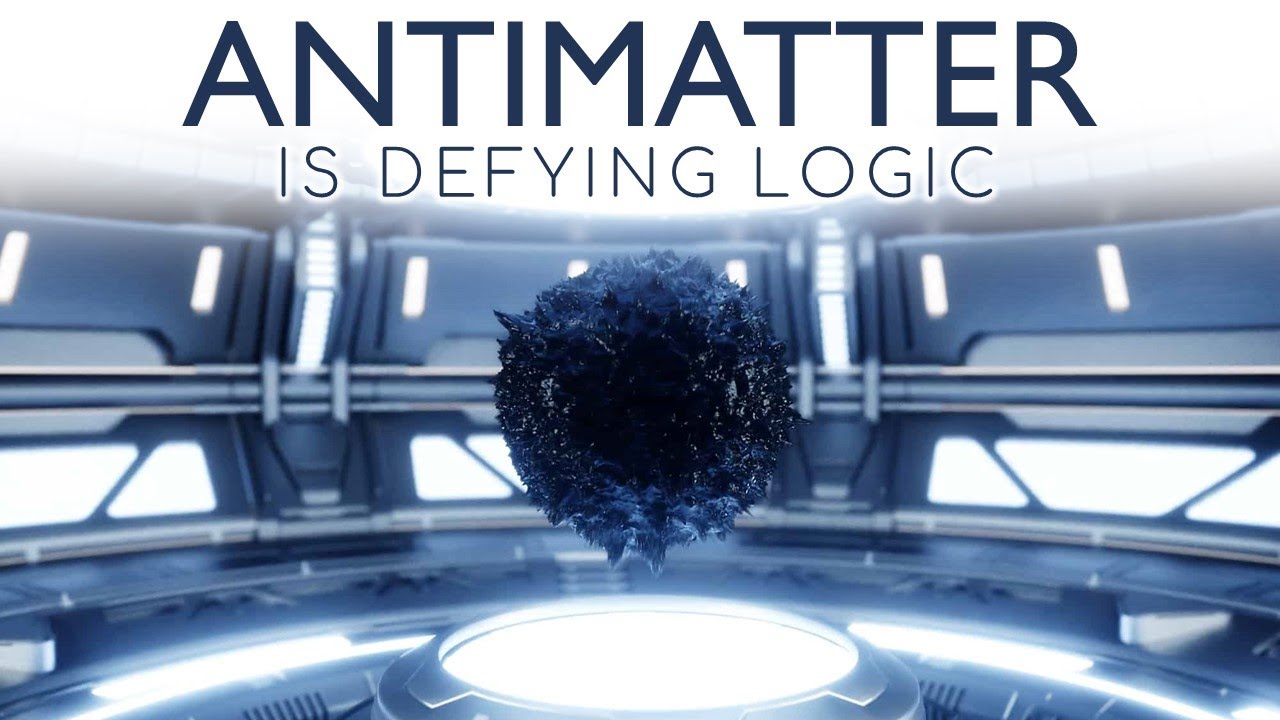
What's Happening With Antimatter at CERN? Scientists Are Stumped Again

Cosmology Lecture 8
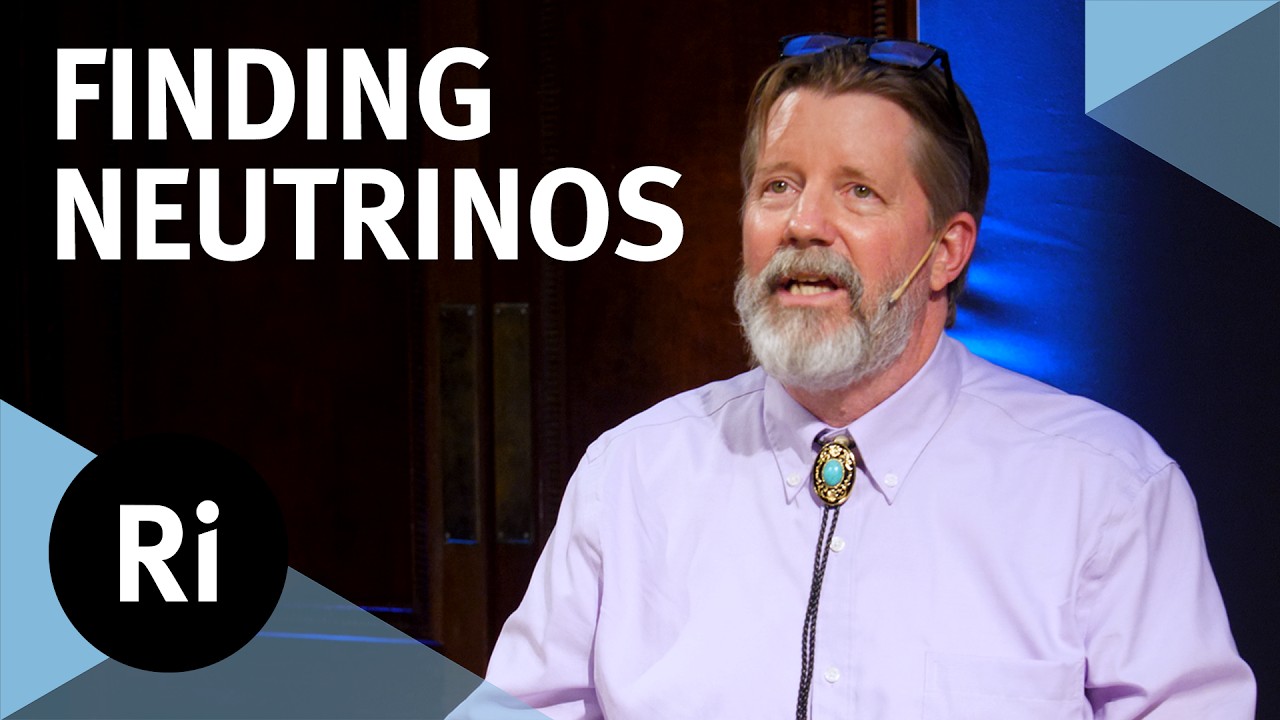
The ghost particle: searching for the mysterious neutrino - with James Riordon
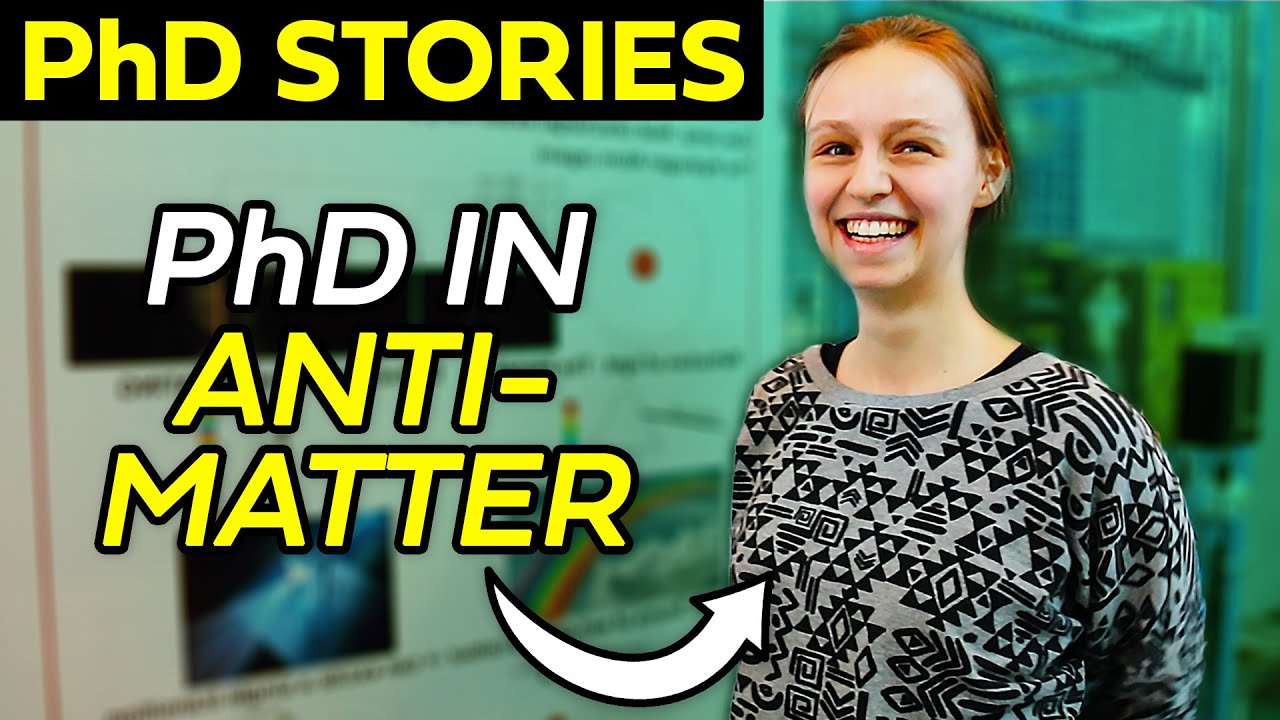
What's it like doing a PhD at CERN?
5.0 / 5 (0 votes)
Thanks for rating: