8. Radioactive Decay β Modes, Energetics, and Trends
TLDRThe lecture delves into the fundamentals of radioactive decay, exploring various decay mechanisms such as alpha, beta, and positron decay. It explains the differences between these processes, their implications for nuclear stability, and how they are represented in decay diagrams. The discussion also touches on the historical context of neutrino discovery and the practical applications of radioactive decay in fields like medical imaging and materials science, exemplified by positron annihilation spectroscopy for detecting atomic defects.
Takeaways
- π Radioactive decay is a fundamental concept in nuclear physics, with various decay modes such as alpha, beta, positron emission, and electron capture.
- π Alpha decay involves the emission of a helium nucleus (two protons and two neutrons), resulting in a decrease in both atomic number and mass number of the parent nucleus.
- π Beta decay, specifically beta-minus decay, involves the transformation of a neutron into a proton within the nucleus, accompanied by the emission of an electron and an antineutrino.
- π― In beta decay, the emitted electrons (beta particles) do not have the same energy as the Q value due to the conservation of momentum, leading to a spectrum of kinetic energies.
- π« Positron decay (or beta-plus decay) occurs when a proton is converted into a neutron, emitting a positron (the antimatter counterpart of an electron) and a neutrino.
- π§ͺ Electron capture is a process where a nucleus captures an inner orbital electron, effectively converting a proton into a neutron and reducing the atomic number by one.
- π Decay diagrams are graphical representations that show the parent nucleus and daughter nucleus, along with the energies and probabilities associated with different decay modes.
- π The stability of nuclei is influenced by the balance of protons and neutrons, with even-even nuclei being more stable than odd-odd nuclei.
- π¬ The detection and measurement of neutrinos are crucial for understanding various nuclear processes and astronomical events, with detectors like Kamiokande in Japan being used for this purpose.
- 𧬠Positron annihilation spectroscopy (PAS) is a technique used to probe the defects in materials by analyzing the lifetime of positrons before they annihilate with electrons.
- βοΈ The danger posed by different types of radioactive decay depends on the energy and charge of the emitted particles, as well as whether the exposure is internal or external to the body.
Q & A
What is the significance of the y-axis in a decay diagram?
-In a decay diagram, the y-axis represents the order of increasing energy (first imaginary y-axis) and atomic number (second imaginary y-axis), which helps in interpreting the diagram and determining how to read and write them.
What happens during alpha decay?
-During alpha decay, a parent nucleus with atomic number Z and mass number A transforms into a helium nucleus, which consists of 2 protons and 2 neutrons, and a daughter nucleus with Z minus 2 protons and A minus 4 total nucleons.
Why is the final state of an alpha decayed daughter nucleus drawn to the left on a decay diagram?
-The final state of an alpha decayed daughter nucleus is drawn to the left on a decay diagram because it represents a decrease in both atomic number (Z) and mass number (A), indicating a move towards a more stable state with lower energy.
What is the difference between a beta particle and an electron in terms of nomenclature?
-A beta particle and an electron are physically identical, but the nomenclature difference is that a beta particle originates from the nucleus, whereas an electron does not.
How does beta decay affect the atomic number and mass number of a nucleus?
-In beta decay, the atomic number of the daughter nucleus increases by one (due to the conversion of a neutron into a proton), but the mass number remains the same as the original nucleus because the total number of nucleons is conserved.
What is electron capture, and how does it differ from beta decay?
-Electron capture is a process where a nucleus captures an electron from one of its inner orbitals, effectively neutralizing a proton and converting it into a neutron. This results in a daughter nucleus with one fewer proton compared to the parent nucleus. Unlike beta decay, which involves the emission of an electron, electron capture involves the absorption of an electron.
What are the conditions required for a nucleus to undergo positron emission?
-A nucleus can undergo positron emission if it has sufficient energy to create a positron. This process typically occurs in nuclei with an excess of protons compared to neutrons.
How does neutron emission differ from other types of radioactive decay?
-Neutron emission is a process where a nucleus emits one or more neutrons to become more stable. Unlike alpha or beta decay, where a new element is formed, neutron emission results in the same element but with a lower mass number, as the total number of nucleons decreases.
What is gamma decay, and what occurs during this type of decay?
-Gamma decay involves the emission of a photon (gamma ray) from the nucleus. This occurs when a nucleus is in an excited state and transitions to a lower energy state. Unlike other forms of decay, the atomic number and mass number remain unchanged, as the decay involves only a change in energy state.
Why do odd-odd nuclei tend to be less stable than even-even nuclei?
-Odd-odd nuclei, which have an odd number of protons and an odd number of neutrons, tend to be less stable because their nuclear shells are not fully occupied. Nucleons (protons and neutrons) prefer to pair up, and unpaired nucleons in odd-odd nuclei can result in higher energy states and less stability compared to even-even nuclei where the shells are fully occupied.
What is the role of the Q value in radioactive decay, and how is it determined?
-The Q value represents the energy released in a decay process. It is determined by the difference in mass between the parent nucleus and the daughter nucleus (along with any emitted particles), and it is usually expressed in mega-electron volts (MeV). The Q value is a key parameter in understanding the energy dynamics of radioactive decay.
Outlines
π Introduction to Radioactive Decay
The video begins with an introduction to radioactive decay, emphasizing its significance in the field of nuclear physics. The lecturer, Michael Short, outlines the general concept of decay and introduces the idea of creating a generalized decay diagram to understand the different decay processes. He also humorously mentions his one-year-old son's artistic intervention on a previous diagram, setting a light-hearted tone for the lecture. The audience is encouraged to participate by suggesting types of radioactive decay, starting with alpha decay.
π₯ Alpha Decay and Its Characteristics
The paragraph delves into alpha decay, describing it as the process where a helium nucleus is emitted from a parent nucleus, resulting in a daughter nucleus with two fewer protons and four fewer total nucleons. The decay diagram for alpha decay is depicted as moving to the left, signifying a decrease in atomic number and energy. The lecture continues with an exploration of beta decay, highlighting the emission of an electron (or beta particle) from the nucleus and the accompanying release of an anti-neutrino.
π« Electron Capture and Positron Emission
The lecture moves on to discuss electron capture, where a nucleus captures an electron from an inner orbital, effectively neutralizing a proton and leading to a daughter nucleus with one fewer proton. Positron emission is also covered, where a positron (the anti-matter equivalent of an electron) is emitted, resulting in the same daughter nucleus as in electron capture but through a different process. The conditions necessary for positron emission are briefly touched upon, with a promise to revisit the topic later in the course.
π Neutron Emission and Gamma Decay
The paragraph covers neutron emission, where a neutron is released from an unstable nucleus, leading to a daughter nucleus with one less total nucleon. This is illustrated on the decay diagram by a downward movement, indicating a decrease in mass number. Gamma decay is introduced as the emission of a photon from the nucleus, which doesn't change the nucleus's identity but rather transitions it to a lower energy state. The paragraph concludes with a discussion of the generalized decay diagram, which encompasses all the decay types discussed.
𧬠Decay Probabilities and Half-Life
The lecture explores the probabilities associated with different decay processes, explaining that these are typically measured due to the complexity of calculating them from first principles. The importance of half-life in understanding decay processes is mentioned, with examples provided to illustrate how half-life can vary for different decay modes of the same isotope. The concept of decay hindrance is introduced, explaining that certain energy transitions are more probable than others, leading to variations in decay patterns.
π Decay Diagram Reading and Alpha Decay Calculation
The paragraph focuses on how to read decay diagrams, including understanding the parent and daughter nuclei, energy levels, and the Q value for a reaction. The calculation for the kinetic energy of an alpha particle during alpha decay is derived using conservation laws for mass, energy, and momentum. The lecture concludes with a discussion of the different decay modes of isotopes like uranium-235 and thorium-231, and the probabilities associated with each mode.
π Beta Decay and Its Energy Spectrum
Beta decay is discussed in detail, highlighting the continuous energy spectrum observed for beta particles, which led to the hypothesis of the neutrino. The lecture touches on the conservation of energy in beta decay and the subsequent gamma transitions that often follow. The use of beta decay in dating through carbon-14 is mentioned, along with its application in medical imaging using technetium-99m and the risks associated with cobalt-60.
β¨ Positrons and Their Applications in Materials Science
The paragraph introduces positron decay, explaining the energy requirements and the relationship between the Q value, mass, and kinetic energy. Positron annihilation spectroscopy (PAS) is explored as a technique to study defects in materials by analyzing the lifetime of positrons before they annihilate with electrons. The lecture concludes with a discussion of how PAS can be used to determine the size and number of vacancies and defects in materials, which is crucial for understanding radiation damage.
βοΈ The Hazards of Different Types of Radioactive Decay
The final paragraph addresses the question of which type of radioactive decay is the most dangerous. It discusses the hazards of alpha, beta, and gamma decay when ingested or when external sources are involved. The paragraph also humorously recounts a hypothetical scenario where one must choose how to handle different types of radioactive 'cookies,' highlighting the different shielding and penetration capabilities of each decay type.
Mindmap
Keywords
π‘Radioactive Decay
π‘Alpha Decay
π‘Beta Decay
π‘Positron Decay
π‘Electron Capture
π‘Gamma Decay
π‘Half-Life
π‘Nuclide
π‘Nuclear Stability
π‘Binding Energy
π‘Neutrino
Highlights
The lecture introduces the concept of radioactive decay and its significance in the field.
The general decay equation is discussed, providing a foundation for understanding specific decay processes.
A generalized decay diagram is developed to illustrate the different decay processes nuclei can undergo.
Alpha decay is explained, detailing the transformation of a parent nucleus into a daughter nucleus with a helium nucleus.
Beta decay is introduced, highlighting the emission of an electron from the nucleus and the resulting change in atomic number.
Electron capture is discussed, explaining how a nucleus captures an electron from its inner orbitals, effectively neutralizing a proton.
Positron emission is described, contrasting it with electron capture and detailing the conditions required for its occurrence.
The concept of gamma decay is introduced, explaining the emission of a photon from the nucleus as a result of an excited state.
The lecture touches on the stability of even-even versus odd-odd nuclei, explaining the concept of nuclear shells and their role in stability.
Potassium-40 is highlighted as an isotope that undergoes multiple decay mechanisms, serving as a comprehensive example of decay processes.
The decay diagram for dysprosium-151 is mentioned as an example of a simple decay process involving only alpha decay.
The lecture explains how decay diagrams are read, including the significance of axes representing energy and atomic number.
The Q value is defined and its role in calculating the energy of alpha particles is discussed.
The concept of half-life is introduced, providing a measure of the stability of radioactive isotopes.
The lecture addresses the detection and measurement of neutrinos, explaining the theoretical basis and experimental methods.
Positron annihilation spectroscopy is introduced as a method for probing material defects using anti-matter.
The dangers of different types of radioactive decay are discussed, with emphasis on the risks associated with internal versus external exposure.
Transcripts
Browse More Related Video

Alpha Decay, Beta Decay, Gamma Decay - Electron Capture, Positron Production - Nuclear Chemistry

Alpha Particles, Beta Particles, Gamma Rays, Positrons, Electrons, Protons, and Neutrons

10. Radioactive Decay Continued
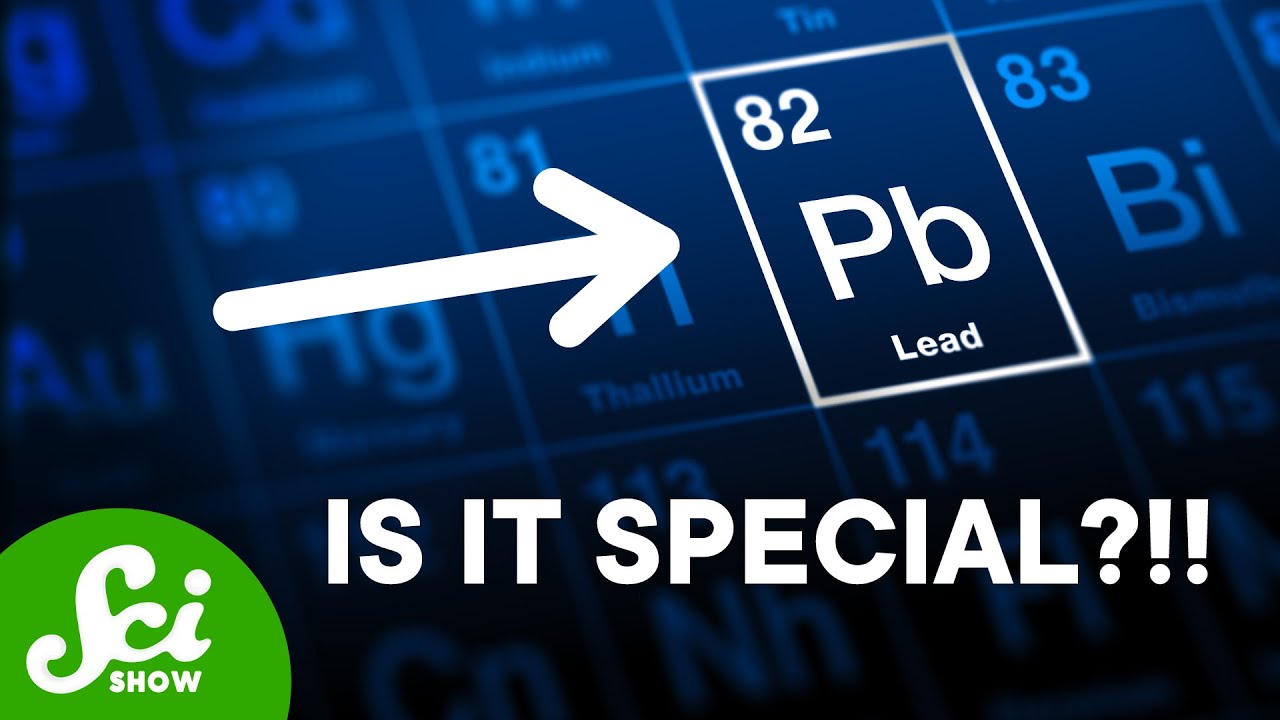
Why Does Everything Decay Into Lead
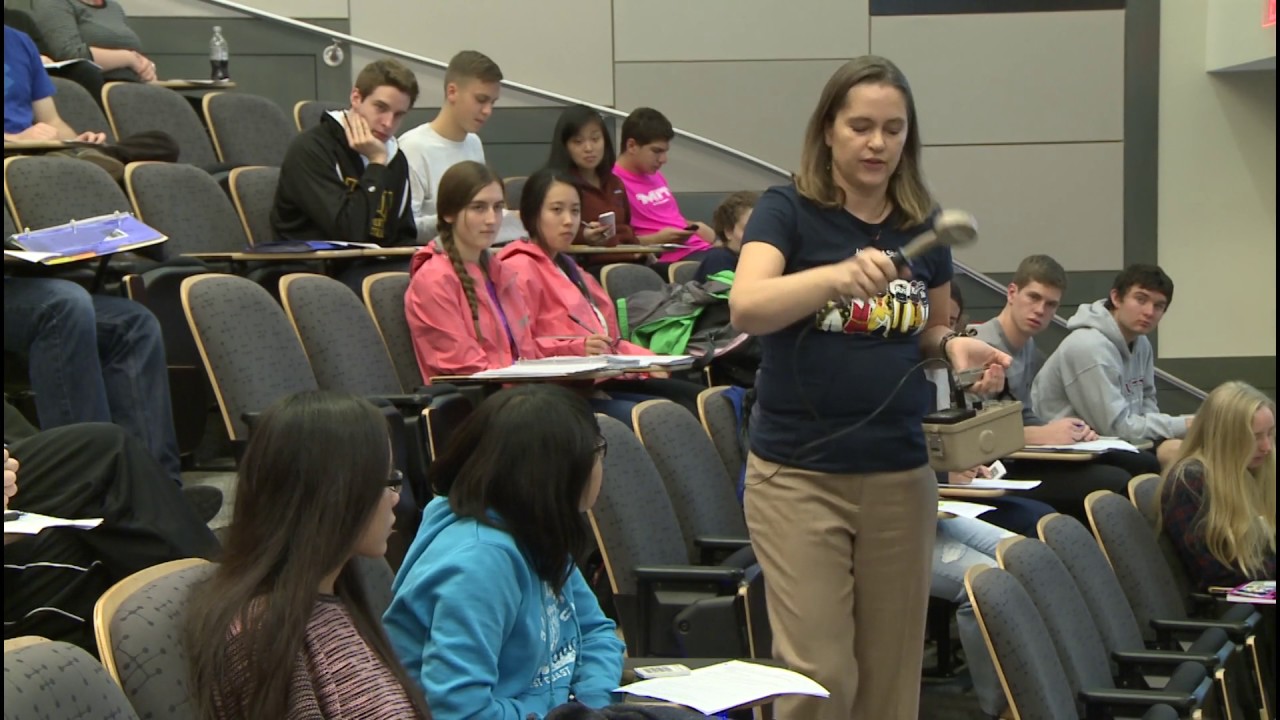
31. Nuclear Chemistry and Chemical Kinetics
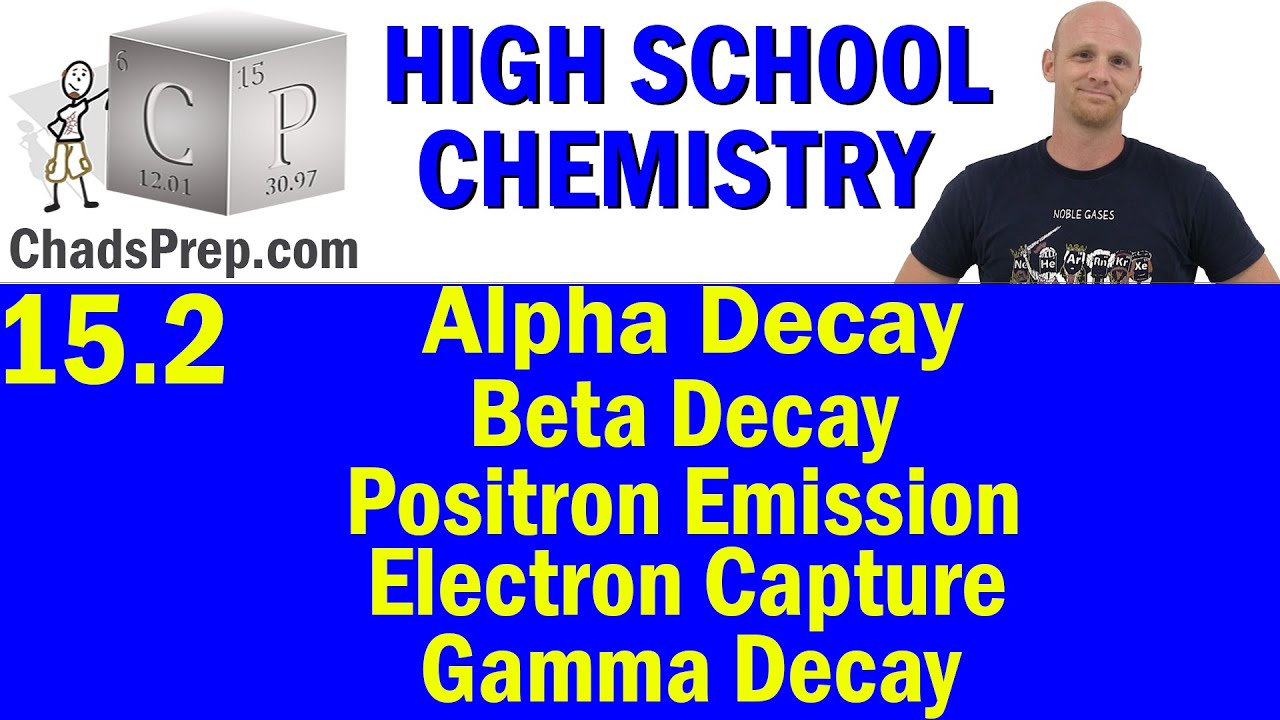
15.2 Routes of Nuclear Decay, Fission, and Fusion | High School Chemistry
5.0 / 5 (0 votes)
Thanks for rating: