Nuclear Reactions, Radioactivity, Fission and Fusion
TLDRProfessor Dave's video delves into the fundamentals of nuclear reactions and the forces governing them, emphasizing the electromagnetic force's role in chemistry and distinguishing it from the strong and weak nuclear forces that operate at the atomic nucleus level. The video explains the processes of nuclear decay and transmutation, the discovery of radioactivity, and the types of radiation, including alpha, beta, positron, and gamma emissions. It also discusses the reasons behind nuclear instability, the factors contributing to it, and the various decay processes that lead to a stable nucleus. The potential energy release from nuclear reactions is highlighted, touching on their applications in atomic bombs and the promise of nuclear fusion for renewable energy, urging viewers to consider their role in technological advancements.
Takeaways
- π The electromagnetic force is responsible for chemical phenomena, including the formation of atoms, chemical bonds, and molecules.
- π There are four fundamental forces in the universe: gravity, electromagnetism, the strong nuclear force, and the weak nuclear force, each operating on different scales.
- π« The strong nuclear force keeps the atomic nucleus together, preventing protons from repelling each other, while the weak nuclear force facilitates nuclear decay and transmutation of elements.
- π’ Henri Becquerel's discovery of radioactivity in 1896 marked the beginning of understanding atomic radiation, which consists of three types of particles: alpha, beta, and gamma.
- π§ Nuclear reactions involve changes to the fundamental particles in an atom's nucleus, leading to a change in the element's identity, unlike chemical reactions which only rearrange valence electrons.
- π’ Nuclide symbols represent the atomic number (protons) and mass number (protons + neutrons), and are used to notate nuclear reactions, ensuring conservation of atomic numbers and mass numbers.
- π₯ Nuclear decay occurs due to instability in the nucleus, which can be caused by being too large, having an imbalanced number of protons or neutrons, or an unfavorable neutron-to-proton ratio.
- π Radioactive decay series involve multiple emissions that eventually lead to a stable nucleus, such as uranium-238 decaying into lead-206.
- 𧬠Radiation can be harmful to biological organisms as it can cause mutations by damaging DNA molecules, but natural radioactive isotopes decaying in the body are usually repaired by enzymes.
- β³ Radioactive materials are characterized by their half-life, the time taken for half of the material to decay, and understanding nuclear processes can lead to harnessing their energy potential.
Q & A
What is the electromagnetic force responsible for?
-The electromagnetic force is responsible for the entirety of chemical phenomena, including the formation of atoms, chemical bonds, molecules, and the reactivities of different substances.
What are the four fundamental forces in the universe?
-The four fundamental forces in the universe are gravity, electromagnetism, the strong nuclear force, and the weak nuclear force.
What keeps the nucleus of an atom together?
-The strong nuclear force keeps the nucleus of an atom together, preventing the positively charged protons from pushing each other away and splitting up the nucleus.
What is the role of the weak nuclear force in nuclear processes?
-The weak nuclear force facilitates nuclear decay, which involves changes in the nucleus of an atom, often leading to the transmutation of elements.
Who discovered radioactivity and how did they discover it?
-Henri Becquerel discovered radioactivity in 1896 when he noticed that photographic plates had bright spots when exposed to uranium minerals.
What are the three types of radiation discovered by Becquerel and how are they named?
-The three types of radiation are alpha particles, beta particles, and gamma particles. They were named with Greek letters at the time of discovery and their identities were discovered later.
How can we represent different particles in nuclear reactions?
-Particles can be represented using symbols or abbreviations such as helium for alpha particles, e or beta for electrons, p for protons, n for neutrons, and gamma for photons of light.
What is the process of transmutation in nuclear reactions?
-Transmutation is the process in which an atom changes from one element to another due to the emission of particles such as alpha or beta particles during nuclear decay.
Why do unstable nuclei undergo radioactive decay?
-Unstable nuclei undergo radioactive decay due to instabilities in the nucleus, such as being too large, having an improper number of protons or neutrons, or having an imbalanced neutron to proton ratio.
What is a radioactive decay series?
-A radioactive decay series is a sequence of multiple emissions that an unstable nucleus undergoes to generate a stable nucleus, such as uranium-238 eventually becoming lead-206.
How do we detect radiation and what is the concept of half-life?
-We detect radiation using devices like Geiger counters. The half-life of a radioactive material is the time it takes for it to be depleted to half its original amount, with each successive half-life reducing the amount further.
How does the concept of nuclear energy relate to Einstein's equation E=mc^2?
-Nuclear energy involves the conversion of matter directly into energy, as described by Einstein's equation E=mc^2. This equation shows that matter is extremely dense energy, and even a small amount of mass can be converted into a tremendous amount of energy.
Outlines
π Introduction to Nuclear Reactions and Forces
This paragraph introduces the concept of nuclear reactions and the fundamental forces in the universe. It begins by explaining the role of the electromagnetic force in chemical phenomena, including the formation of atoms, chemical bonds, and molecules. The script then outlines the four fundamental forces: gravity, electromagnetism, the strong nuclear force, and the weak nuclear force, detailing their respective scales of operation and their significance in the cosmos. The strong nuclear force is described as the force that holds the atomic nucleus together, while the weak nuclear force facilitates nuclear decay, leading to the transmutation of elements. The paragraph also touches on the historical discovery of radioactivity by Henri Becquerel and the identification of three types of radiation: alpha, beta, and gamma particles.
π¬ Understanding Nuclear Decay and Stability
This paragraph delves into the reasons behind nuclear decay, explaining that it occurs due to instabilities within the atomic nucleus. It discusses how the strong nuclear force, mediated by mesons, keeps protons and neutrons together but weakens with distance, leading to instability in larger nuclei. The script then explores the shell model of the nucleus, highlighting the concept of 'magic numbers' that correspond to nuclear stability. It also addresses the ideal neutron-to-proton ratios for nuclei and how decay processes like alpha emission, beta emission, positron emission, electron capture, and gamma emission help nuclei adjust to achieve stability. The paragraph concludes by discussing the potential causes of various decay processes based on the nucleus's condition.
β’οΈ Radiation, Radioactivity, and Nuclear Energy
The final paragraph discusses the nature of radiation and its effects on biological organisms, emphasizing the potential harm caused by high-energy particles to cells and DNA. It mentions the natural radioactive isotopes present in elements like carbon, hydrogen, and oxygen, and the body's ability to repair DNA damage. The script then introduces the concept of half-life and its significance in understanding the decay process. It transitions into the application of nuclear processes, highlighting the conversion of matter into energy as described by Einstein's equation, e=mc^2. The paragraph concludes by reflecting on the historical use of nuclear energy in atomic bombs and the potential of nuclear fusion as a powerful and renewable energy source for future societal advancement.
Mindmap
Keywords
π‘Nuclear Reactions
π‘Electromagnetic Force
π‘Strong Nuclear Force
π‘Weak Nuclear Force
π‘Radioactivity
π‘Alpha Particle
π‘Beta Particle
π‘Gamma Particle
π‘Nuclide Symbols
π‘Half-Life
π‘Einstein's Equation (E=mc^2)
Highlights
The electromagnetic force is responsible for all chemical phenomena, including the formation of atoms, chemical bonds, and molecules.
There are four fundamental forces in the universe: gravity, electromagnetism, strong nuclear force, and weak nuclear force.
Chemical reactions involve the rearrangement of valence electrons, while nuclear reactions involve changes to the fundamental particles in the nucleus.
Henri Becquerel's discovery of radioactivity in 1896 marked the beginning of understanding atomic nuclei and their transformations.
Radiation was found to be composed of three types of particles: alpha particles, beta particles, and gamma particles, each with distinct properties and behaviors.
Nuclear reactions can be notated using specific symbols and atomic numbers, allowing for the tracking and prediction of nuclear decay processes.
Nuclear instability can arise from a nucleus being too large, having an imbalance in the number of protons or neutrons, or an unfavorable neutron-to-proton ratio.
The shell model of the nucleus suggests that there are 'magic numbers' of nucleons that correspond to a special type of stability.
Radioactive decay can result in the transmutation of elements, changing one atom into another through various types of emissions or fission.
Radiation can be harmful to biological organisms as it can cause damage to cells and potentially alter genetic information.
Half-life is a concept used to describe the time it takes for a radioactive substance to decay to half of its original amount.
Einstein's equation, E=mc^2, demonstrates that matter is a highly dense form of energy, which can be released through nuclear reactions.
Nuclear power, both through fission and fusion, has the potential to provide massive amounts of energy and could be key in solving renewable energy issues.
The development of technologies such as Geiger counters has been crucial in detecting and managing radiation levels.
Unstable isotopes naturally occur in many elements, including carbon, hydrogen, and oxygen, and decay continuously, albeit at varying rates.
The study and understanding of nuclear processes can lead to advancements in technology and energy solutions, potentially benefiting society greatly.
Transcripts
Browse More Related Video
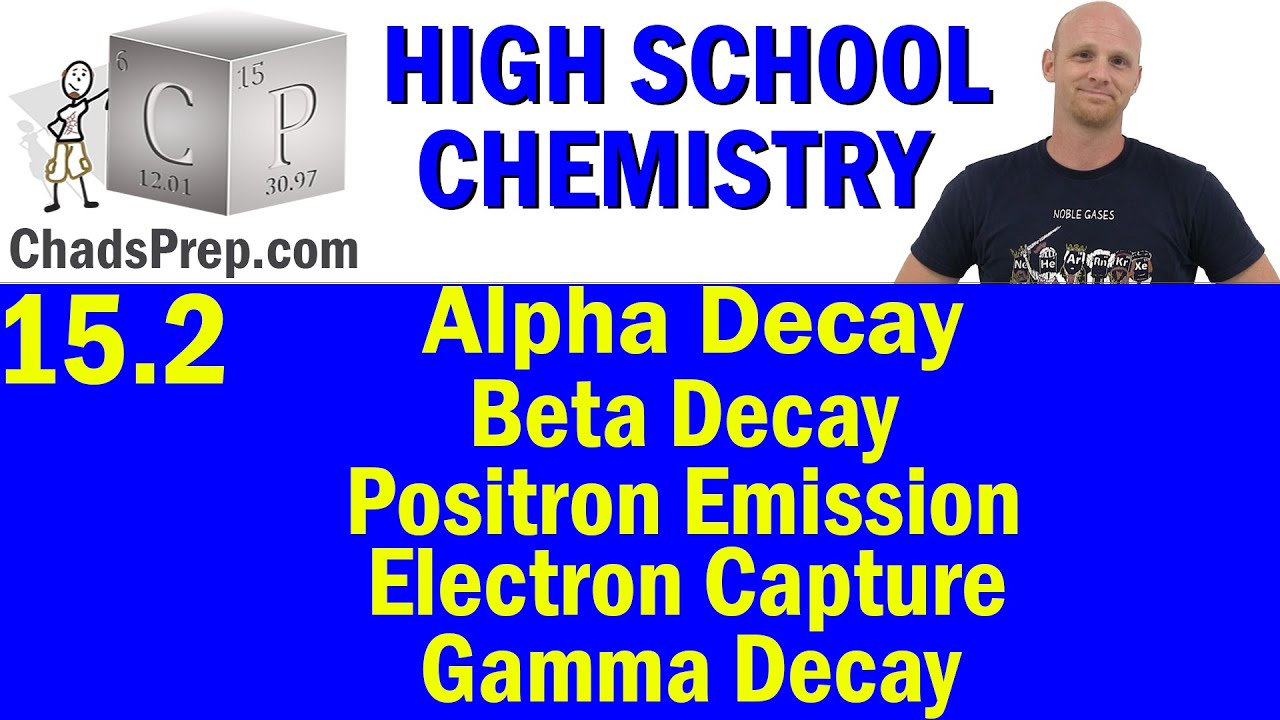
15.2 Routes of Nuclear Decay, Fission, and Fusion | High School Chemistry
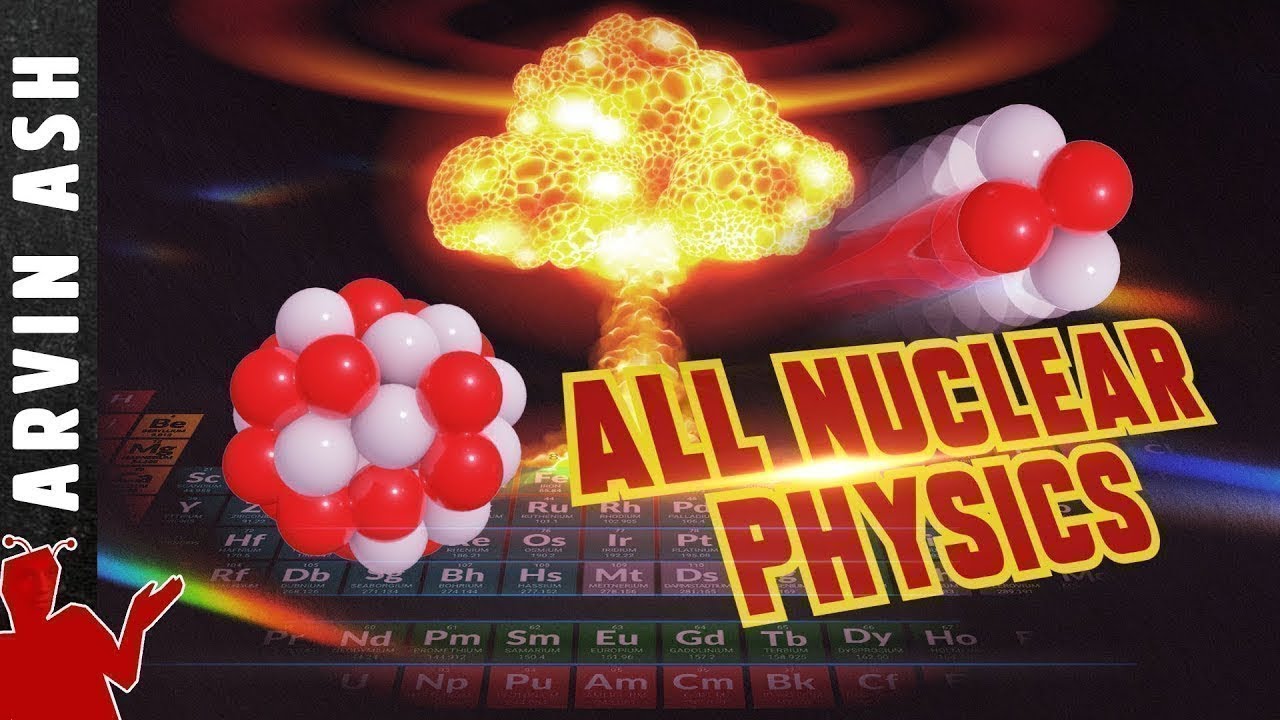
ALL Nuclear Physics Explained SIMPLY

15.1 Introduction to Nuclear Chemistry | High School Chemistry

Nuclear Physics: Crash Course Physics #45
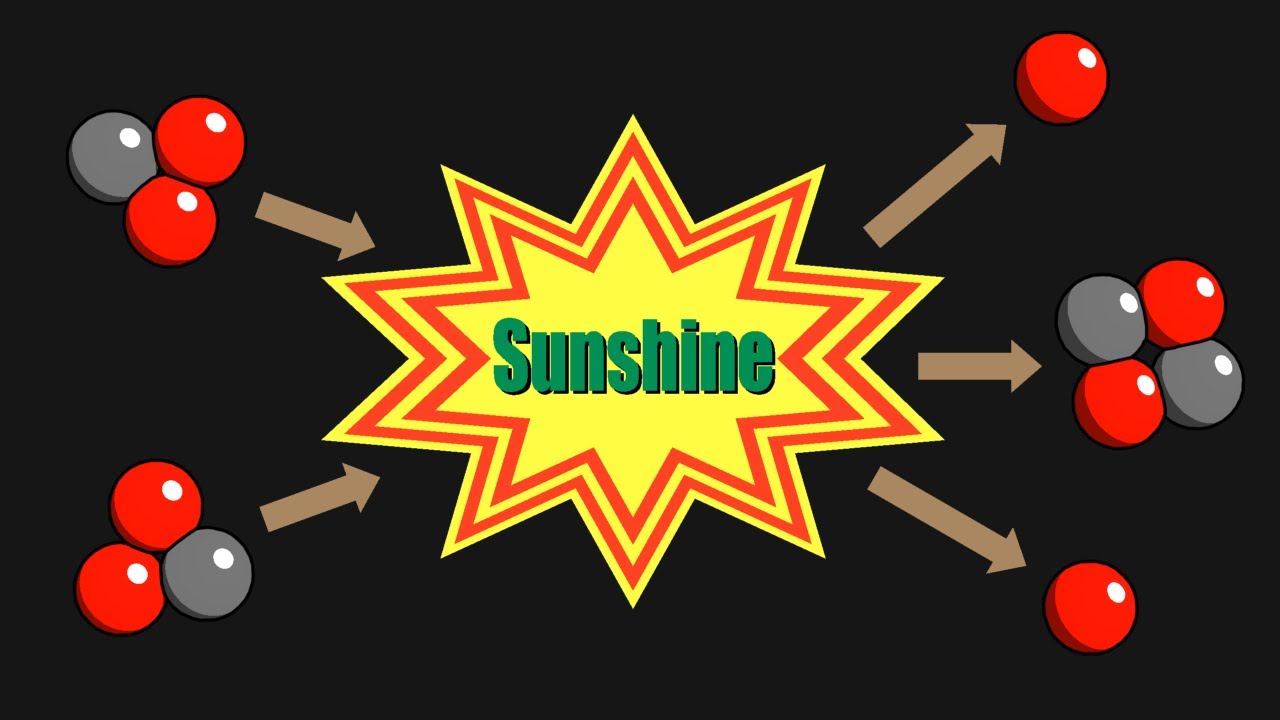
How the Sun Shines: The Nuclear Reactions that Power the Sun
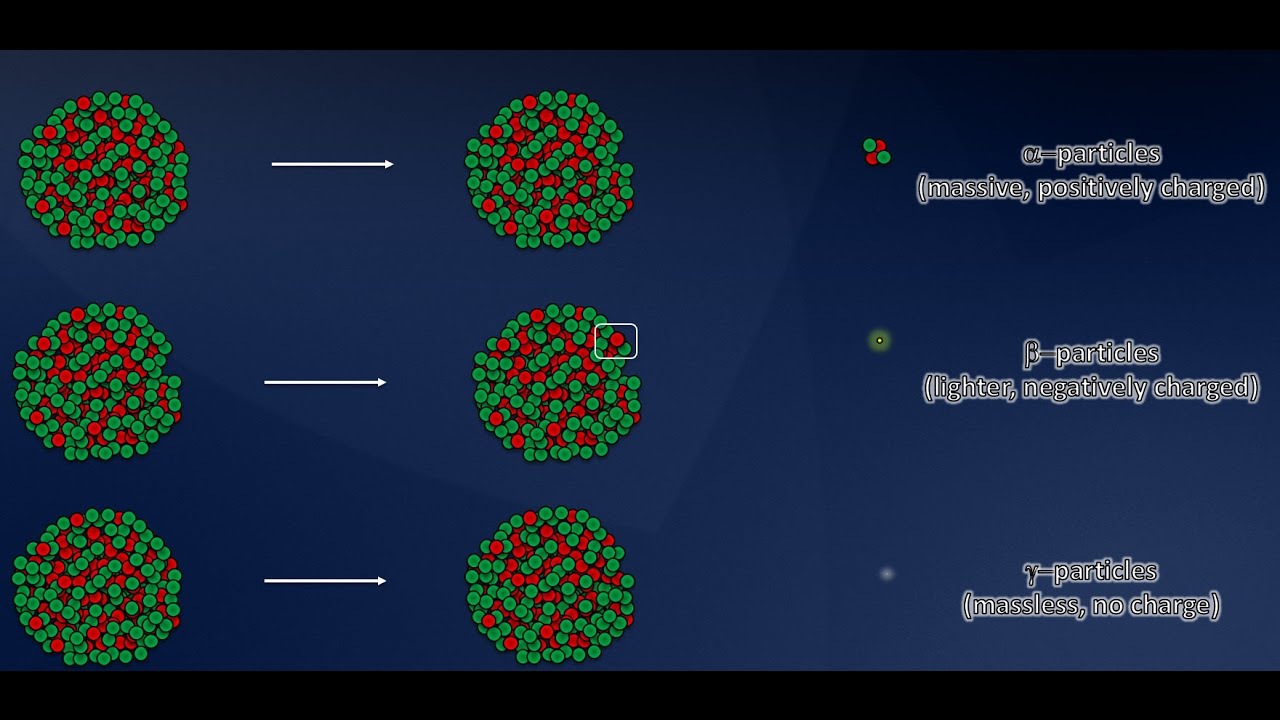
A Brief Introduction to Alpha, Beta and Gamma Radiation
5.0 / 5 (0 votes)
Thanks for rating: