Ultrasound Physics with Sononerds Unit 10
TLDRThis educational video script delves into the concepts of axial and lateral resolution in ultrasound imaging, crucial for image accuracy. It explains how axial resolution, determined by spatial pulse length, and lateral resolution, influenced by beam width, affect the clarity of ultrasound images. The script covers the physics behind these resolutions, their clinical implications, and how modern ultrasound equipment, including electronic focusing, can optimize them for better diagnostic images.
Takeaways
- π Axial and lateral resolution are critical in ultrasound imaging for accurately displaying details within the body.
- π Axial resolution refers to the ability to display two reflectors parallel to the sound beam accurately and is determined by the spatial pulse length (SPL).
- π Lateral resolution is the capability to image reflectors side by side or perpendicular to the sound beam and is influenced by the beam width.
- π Resolution is not adjustable with a simple knob on the machine; it requires understanding the physics behind it, including the relationship between frequency, wavelength, and SPL.
- π’ The formula for axial resolution is SPL divided by 2, indicating that a smaller SPL results in better resolution.
- π¬ High-frequency transducers and fewer cycles per pulse improve axial resolution, while low-frequency transducers degrade it.
- π Lateral resolution is best at the focus of the beam and worsens as the beam diverges in the far field.
- π§ Electronic focusing, a feature of modern ultrasound machines, allows sonographers to adjust the focus to optimize image quality for specific anatomical structures.
- π οΈ Focusing techniques, including the use of lenses and curved elements, affect the beam's diameter, near field, divergence, and focal zone size.
- π High-frequency transducers provide better resolution in the near field but attenuate quickly, limiting their imaging depth.
- π The sonographer must balance the choice of transducer frequency to achieve the best axial and lateral resolution while maintaining adequate imaging depth.
Q & A
What is the main focus of Unit 10 in the provided video script?
-Unit 10 focuses on axial and lateral resolution in the context of ultrasound imaging, explaining how these types of resolution affect the accuracy and detail of the images produced.
What does 'resolution' refer to in the context of ultrasound imaging?
-In ultrasound imaging, 'resolution' describes the ability to display detail accurately. Higher resolution means a more accurate display, which is preferred for image accuracy.
What are the two main types of resolution discussed in the script?
-The two main types of resolution discussed are axial resolution, which determines how accurately a system can display two reflectors parallel to the sound beam, and lateral resolution, which determines the accuracy of side-by-side structures being displayed.
How is axial resolution defined in the script?
-Axial resolution is defined as the machine's capability to accurately image reflectors that are parallel to the sound beam.
What is spatial pulse length (SPL), and how does it relate to axial resolution?
-Spatial pulse length (SPL) is the distance that a pulse takes up in space. Axial resolution is determined by the SPL, specifically, the axial resolution in millimeters is calculated as the SPL divided by 2.
Why is a smaller numerical value for axial resolution considered better?
-A smaller numerical value for axial resolution indicates a smaller minimum distance between two reflectors that need to be parallel to the sound beam to be resolved, thus allowing for more detailed imaging.
How does the number of cycles in a pulse affect axial resolution?
-The number of cycles in a pulse is directly related to axial resolution. More cycles result in a longer spatial pulse length, which leads to a higher numerical value for axial resolution (since it's SPL divided by 2), making it worse. Fewer cycles result in a shorter SPL, improving axial resolution.
What is the relationship between frequency and axial resolution?
-Frequency and axial resolution are inversely related. As frequency increases, the wavelength decreases, leading to a shorter spatial pulse length and thus better (lower numerical value) axial resolution.
How does the use of a lens in a transducer affect the focus of the ultrasound beam?
-A lens in a transducer refracts the sound beam, creating a more narrow focus. This external focus can improve the lateral resolution but is fixed and cannot be adjusted during imaging.
What is the significance of the focus in improving lateral resolution?
-The focus is the point where the ultrasound beam is the narrowest, providing the best lateral resolution. Placing the focus at or just below the area of interest helps to improve the detail and accuracy of the ultrasound image in that region.
Outlines
π Introduction to Axial and Lateral Resolution
The script introduces the concept of resolution in ultrasound imaging, focusing on axial and lateral resolution. It explains that resolution is the ability to display detail accurately, with higher resolution indicating more accurate imaging. The paragraph outlines different types of resolution, including spatial, contrast, temporal, elevational, and axial and lateral resolutions, which are the main focus of the lecture. Axial resolution is defined as the ability to display two reflectors parallel to the sound beam accurately, while lateral resolution is the accuracy of displaying side-by-side structures. The script also mentions that axial resolution depends on spatial pulse length and that lateral resolution depends on beam width, both of which have been previously covered.
π Axial Resolution: Definition and Calculation
This paragraph delves into the specifics of axial resolution, explaining it as the capability of a machine to image reflectors parallel to the sound beam accurately. It discusses how reflectors within the length of the pulse are considered parallel. The paragraph uses an example of orange reflectors to illustrate how axial resolution affects their display. It also introduces the concept of resolved and unresolved reflectors, explaining that accurate display results in resolved reflectors, while inaccuracies lead to unresolved ones. The spatial pulse length (SPL) is identified as a determinant of axial resolution, with a formula provided to calculate it. The average axial resolution for an ultrasound system is given as 0.05 to 0.5 millimeters, emphasizing the importance of a low numerical value for better resolution.
π¬ Understanding Axial Resolution's Dependence on SPL
The script explains the relationship between axial resolution and spatial pulse length (SPL), emphasizing that a smaller SPL results in better axial resolution. It uses an example to illustrate how SPL affects the minimum distance required between two reflectors to be resolved. The paragraph also describes the process of sound wave propagation and reflection, explaining how overlapping echoes from too-close reflectors can lead to unresolved displays. The importance of using high-frequency transducers to improve axial resolution by reducing SPL is highlighted, as well as the inverse relationship between frequency and axial resolution.
π Lateral Resolution: Imaging Reflectors Perpendicular to the Beam
This paragraph introduces lateral resolution, which is the ability to accurately image reflectors that are perpendicular or side by side to the sound beam. It explains how the width of the beam affects the display of these reflectors, with a narrower beam width leading to better lateral resolution. The concept of point spread artifact is introduced, where unresolved reflectors appear wider than they are. The paragraph outlines the formula for calculating lateral resolution, which is the beam width, and emphasizes that a smaller numerical value indicates better resolution.
π Lateral Resolution and Beam Width
The script discusses how lateral resolution is affected by the width of the ultrasound beam and how it changes with depth. It explains that the best lateral resolution is achieved at the focus of the beam, where it is narrowest. The paragraph also covers how the beam width is related to the diameter of the transducer's crystal and how it diverges after the focus, leading to degraded lateral resolution in the far field. The importance of placing the area of interest within the focal zone for optimal imaging is highlighted.
π οΈ Clinical Implications of Axial and Lateral Resolution
This paragraph discusses the clinical implications of axial and lateral resolution, emphasizing the need to balance high-frequency transducers for better resolution with low-frequency transducers for greater imaging depth. It explains that high-frequency transducers improve both axial and lateral resolution but have limited penetration depth, while low-frequency transducers provide deeper imaging but at the cost of resolution. The paragraph encourages sonographers to choose the highest frequency transducer that can visualize the necessary anatomy and to use modern tools to adjust the focus appropriately.
π Focusing Techniques in Ultrasound Imaging
The script introduces focusing techniques used in ultrasound transducers to improve image resolution. It differentiates between lenses, which provide an external fixed focus; curved elements, which offer an internal fixed focus; and electronic focusing, which is adjustable and used in modern machines. The paragraph explains how lenses and curved elements physically alter the sound beam's path to create a more narrow focus, while electronic focusing allows the sonographer to adjust the focus based on the area of interest.
π§ Electronic Focusing and Its Advantages
This paragraph focuses on electronic focusing, highlighting its flexibility and the ability for sonographers to adjust the focus according to the area of interest. It explains that electronic focusing is only possible with multi-element or array transducers, where each element can be activated in a specific pattern to create a focus at the desired depth. The advantages of electronic focusing include improved lateral resolution and the ability to optimize the image for the specific anatomy being examined.
π Effects of Focusing on Beam Behavior
The script concludes with a discussion on the effects of focusing on the behavior of the ultrasound beam. It outlines four effects: the beam diameter in the near field being smaller than the element, the focus moving closer to the transducer which shortens the near zone length, rapid divergence of the beam after the focal zone, and a reduction in the size of the focal zone in both length and diameter. These effects contribute to improved lateral resolution and image quality.
Mindmap
Keywords
π‘Axial Resolution
π‘Lateral Resolution
π‘Spatial Pulse Length (SPL)
π‘Reflectors
π‘Ultrasound Transducer
π‘Frequency
π‘Beam Width
π‘Focus
π‘Point Spread Artifact
π‘Synonyms for Resolution
Highlights
Axial and lateral resolution are critical for accurate ultrasound imaging, determining the clarity of structures parallel and perpendicular to the sound beam, respectively.
Axial resolution is influenced by spatial pulse length, with a formula provided to calculate it, emphasizing its dependence on the number of cycles in a pulse and wavelength.
Lateral resolution is determined by the beam width, with narrower beams offering better resolution for side-by-side structures.
Higher resolution imaging is preferred for increased accuracy, with various types of resolution including spatial, contrast, temporal, and elevational resolution.
The concept of 'resolved' and 'unresolved' reflectors is introduced, illustrating the impact of resolution on the clarity of imaging.
The average axial resolution for an ultrasound system is detailed, providing a benchmark for expected imaging capabilities.
The relationship between spatial pulse length and axial resolution is explored, with a formula for SPL and its impact on imaging explained.
The importance of frequency in axial resolution is discussed, noting that higher frequency transducers improve resolution quality.
Lateral resolution is shown to change with depth due to the natural divergence of the sound beam, affecting image clarity.
The impact of transducer diameter on lateral resolution is highlighted, with smaller diameters providing better resolution at the focus.
Clinical implications of axial and lateral resolution are discussed, guiding sonographers on choosing the right equipment for optimal imaging.
Different focusing techniques used in transducer construction are presented, including lenses, curved elements, and electronic focusing.
Electronic focusing is identified as a modern tool allowing sonographers to adjust the focus for improved image quality.
The effects of focusing on beam characteristics are outlined, detailing changes in the near field, focal zone, and divergence.
Practical advice for sonographers on balancing frequency and transducer choice to optimize both axial and lateral resolution is provided.
A discussion on the importance of placing the focal point correctly to enhance image detail and resolution is included.
Synonyms and alternative names for axial and lateral resolution are presented to familiarize learners with various terminologies used in the field.
The educational content is rounded off with practice questions and answers to reinforce understanding of axial and lateral resolution concepts.
Transcripts
Browse More Related Video
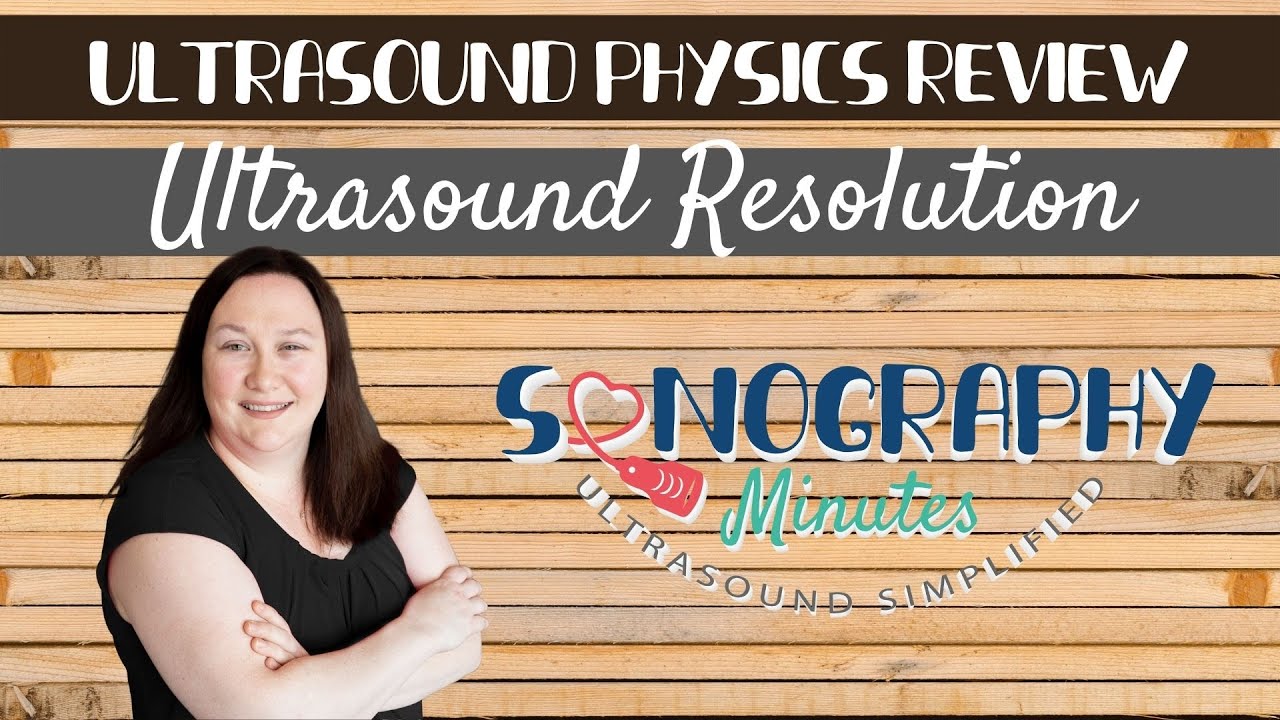
Ultrasound Physics Review | Resolution | Sonography Minutes

Axial Resolution | Ultrasound Physics | Radiology Physics Course #17
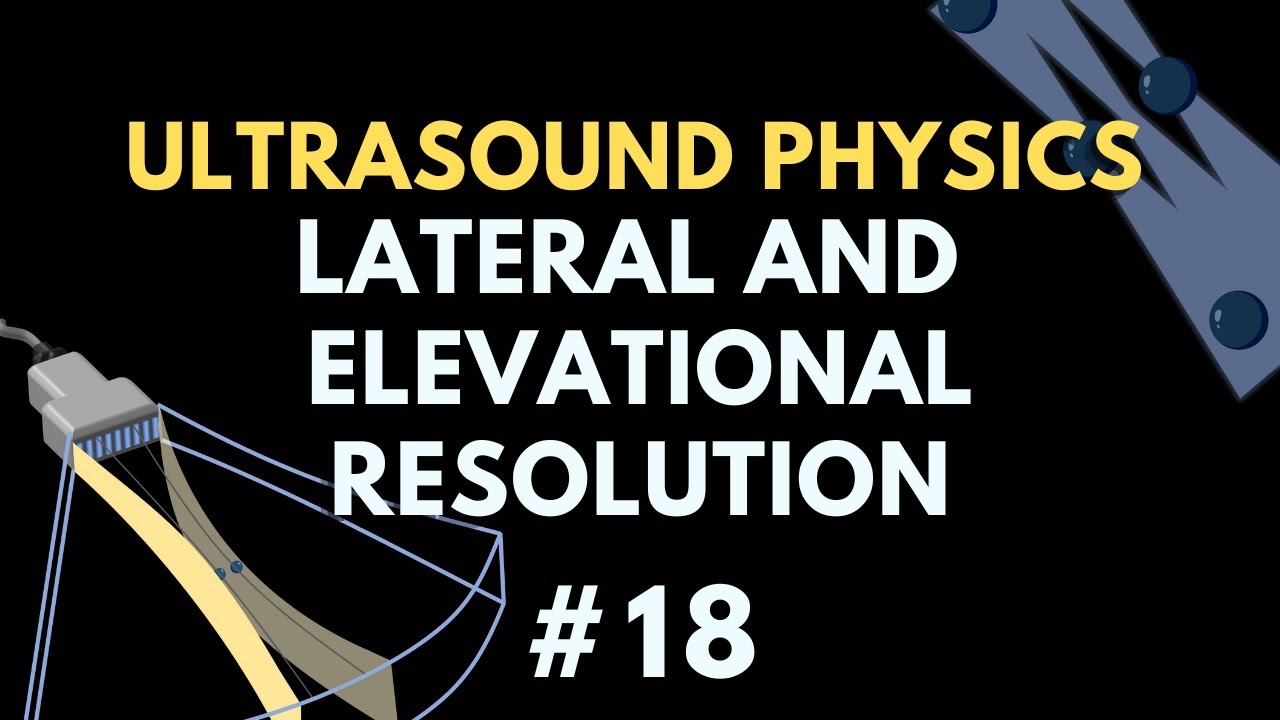
Lateral and Elevational Resolution | Ultrasound Physics | Radiology Physics Course #18

Ultrasound Physics with Sononerds Unit 12b
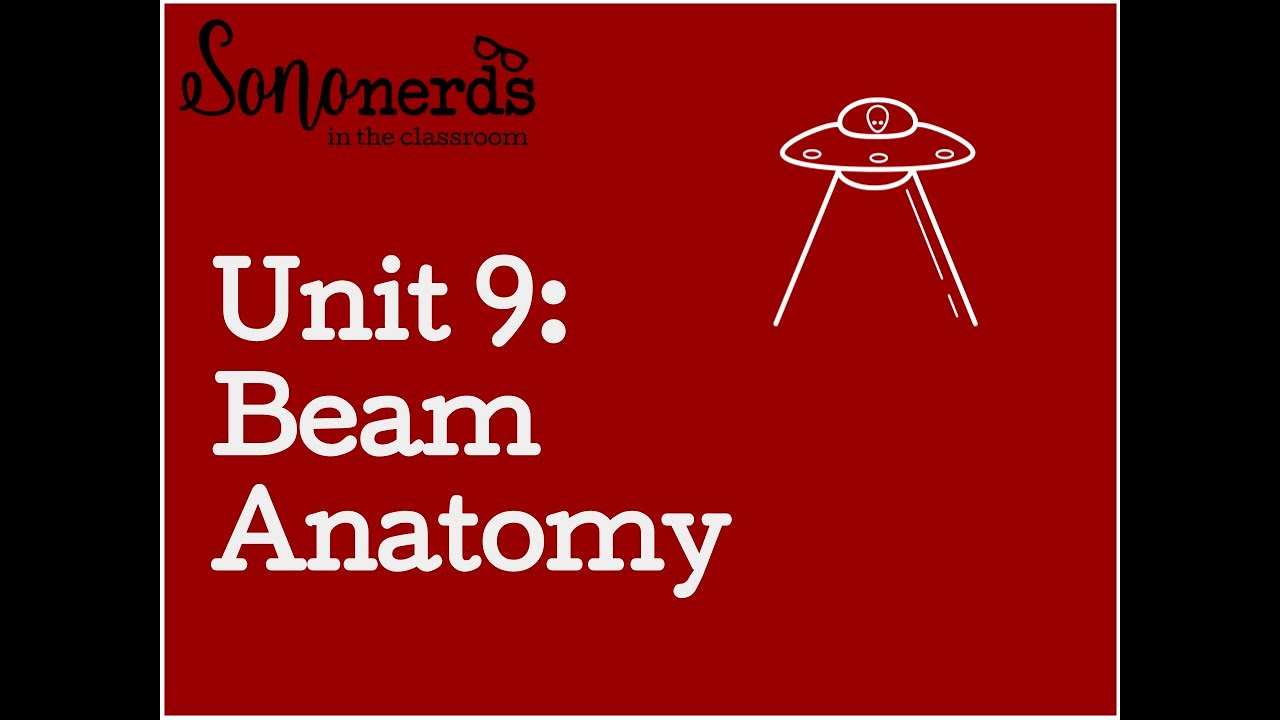
Ultrasound Physics with Sononerds Unit 9
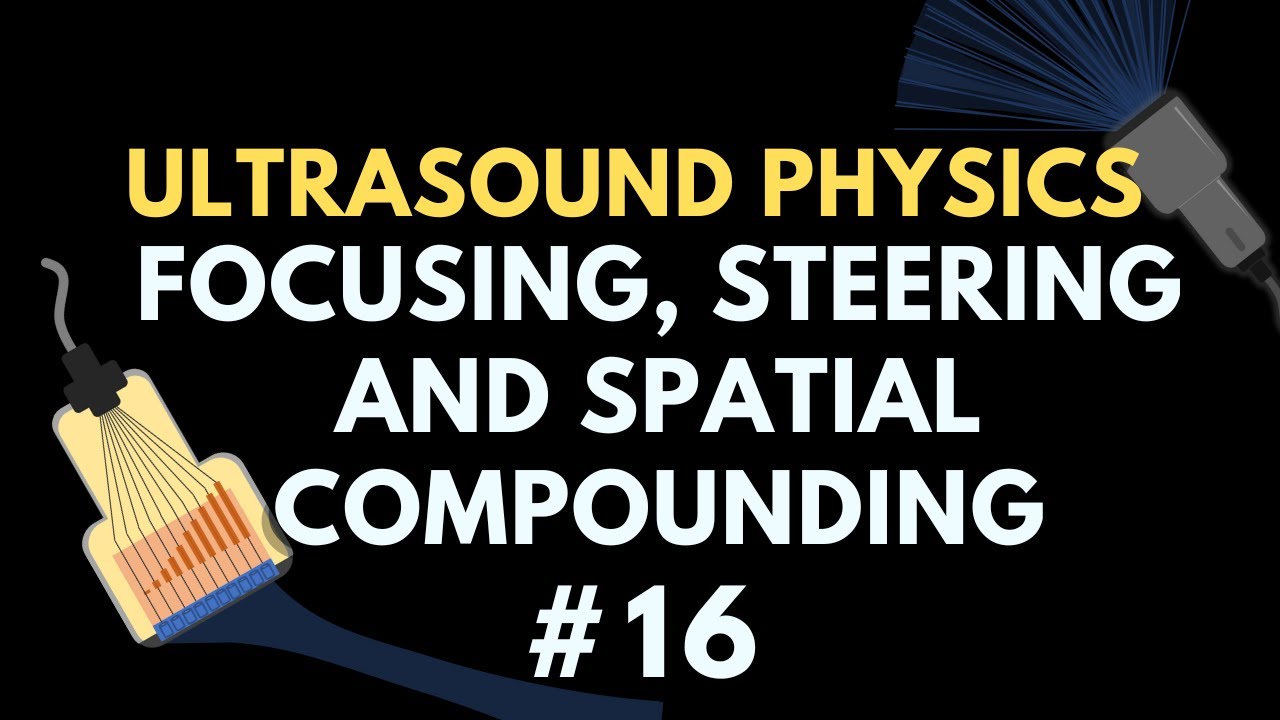
Beam Focusing, Steering and Spatial Compounding | Ultrasound Physics | Radiology Physics Course #16
5.0 / 5 (0 votes)
Thanks for rating: