Phiala Shanahan Public Lecture: The Building Blocks of the Universe
TLDRDr. Fiona Shanahan, an assistant professor of physics at MIT, delivered a public lecture at the Perimeter Institute in Waterloo, Ontario, Canada. She discussed the fundamental question of what everything is made of, delving into the standard model of particle physics that describes the 17 fundamental particles and their interactions. The lecture covered the importance of understanding the standard model to explore new physics beyond it, such as the mysteries of dark matter and dark energy, which together make up about 95% of the universe. Dr. Shanahan highlighted the computational challenges in calculating properties like the mass of a proton and the pressure inside it, and how advancements in supercomputing, machine learning, and quantum computing could potentially unlock new insights into the universe's structure. She also addressed the proton radius puzzle and the quest to reconcile differing experimental measurements. The lecture concluded with a Q&A session, where Dr. Shanahan engaged with the audience on topics ranging from the possibility of new forms of matter to advice for young students interested in theoretical physics.
Takeaways
- 📚 Dr. Fiona Shanahan, an assistant professor of physics at MIT, discusses the fundamental building blocks of the universe, which are believed to be quarks and gluons.
- 🚀 The Standard Model of Particle Physics describes 17 fundamental particles and three of the four fundamental forces, but it does not include gravity.
- 🧠 Dr. Shanahan emphasizes the importance of understanding the Standard Model to identify where it breaks down, which can lead to new discoveries about the universe.
- 💻 High-precision calculations in physics, such as determining the mass of a proton, require powerful supercomputers due to the complexity and time-consuming nature of the computations.
- ✨ Dr. Shanahan highlights the interplay between theory and experiment in physics, noting that both are crucial for advancing knowledge about the universe.
- ⚛️ The pressure distribution within a proton, as calculated using the Standard Model, shows a repulsive pressure at the core and a confining pressure at the edges, with peak pressures exceeding those inside a neutron star.
- ☀️ The Standard Model also explains the nuclear reactions that occur in the Sun, which are responsible for the energy that reaches Earth.
- 🌌 Dark matter, which does not interact with electromagnetic radiation and is thus invisible to our observations, is inferred from its gravitational effects on galaxies and galaxy clusters.
- 🔬 Three main experimental approaches to studying dark matter are collider experiments, direct detection, and indirect detection, each providing different insights into the nature of dark matter.
- 📉 The discrepancy in the measured values of the proton radius from different experimental methods is a significant puzzle in physics, potentially indicating new physics beyond the Standard Model.
- 🔍 Machine learning and quantum computing are among the new computational tools being explored to tackle the complex calculations required to further understand the Standard Model and nuclear physics.
Q & A
What is the topic of Dr. Fiona Shanahan's lecture?
-Dr. Fiona Shanahan's lecture is about the fundamental building blocks of the universe and how they are described by the standard model of particle physics.
What are the two main motivations for studying the standard model of particle physics?
-The two main motivations are to understand how the universe is built from the 17 fundamental building blocks and to understand new physics beyond the standard model.
What are the four fundamental forces described by the standard model?
-The standard model describes three of the four fundamental forces: the strong nuclear force, the electromagnetic force, and the weak nuclear force. It does not describe gravity, which is accounted for by general relativity.
What is the role of the Higgs boson in the standard model?
-The Higgs boson is the last piece of the standard model to be discovered and is responsible for giving particles their mass.
Why is the mass difference between the proton and neutron essential for life as we know it?
-The small mass difference between the proton and neutron is crucial because it allows for the stability of atoms. If the mass difference were reversed, protons could decay, and matter might collapse into black holes or neutron stars, preventing the formation of life.
What is the significance of the pressure distribution inside a proton?
-The pressure distribution inside a proton is significant because it provides insights into the internal dynamics of protons. The pressure at its peak inside a proton is greater than that inside a neutron star, indicating a complex and dynamic structure within the proton.
What are the three main approaches to detecting dark matter?
-The three main approaches to detecting dark matter are collider experiments, where standard model particles are smashed together to potentially create dark matter; direct detection, where a detector on Earth waits for dark matter to interact with it; and indirect detection, which looks for excess standard model particles that could be a result of dark matter annihilation in the universe.
Why is computational power crucial for understanding the standard model of particle physics?
-Computational power is crucial because the calculations required to understand the standard model, especially for complex systems like larger nuclei or dark matter interactions, are extraordinarily demanding and would take an unfeasibly long time with conventional computing methods.
What is the current challenge in unifying the standard model with the theory of general relativity?
-The current challenge is that the standard model and general relativity describe the universe in fundamentally different ways. Unifying these two frameworks into a single, coherent theory that describes all fundamental forces and particles is a major unsolved problem in physics.
What is the role of Moore's Law in the advancement of computational power for physics research?
-Moore's Law predicts an exponential increase in computational power, which is essential for advancing physics research. It suggests that computational capabilities double approximately every two to three years, enabling more complex and precise calculations in particle physics.
How does Dr. Shanahan envision the use of machine learning and quantum computing in the future of theoretical physics?
-Dr. Shanahan sees the potential for machine learning and quantum computing to revolutionize the way complex calculations are performed in theoretical physics. These technologies could allow for more efficient algorithms and tailored computational approaches, potentially leading to breakthroughs in understanding phenomena like dark matter and the proton radius puzzle.
Outlines
🎓 Introduction and Acknowledgements
The video begins with Heather Clark, the executive director of advancement at the Perimeter Institute, welcoming the audience to a public lecture at the Mike Lazaridis Theatre of Ideas in Waterloo, Ontario, Canada. She acknowledges the support of For Women in Science and introduces Dr. Fiona Shanahan, an assistant professor of physics at MIT, who is the evening's speaker. Dr. Shanahan's accomplishments and the significance of the Emmy Noether fellowship program, which supports gender diversity in science, are highlighted.
🌌 The Fundamental Building Blocks of the Universe
Dr. Shanahan's lecture focuses on the fundamental question of what everything is made of. She discusses the historical context and the scientific progress made since ancient times. The current understanding is that matter is composed of atoms, which in turn are made of protons, neutrons, and electrons. Protons and neutrons are themselves composed of quarks held together by gluons, which are the carriers of the strong nuclear force. Dr. Shanahan emphasizes that, as far as current knowledge goes, quarks and gluons are the most fundamental particles in the universe.
🔬 The Standard Model of Particle Physics
The standard model of particle physics is introduced, which describes the interactions of 17 fundamental particles, including quarks, leptons, and force-carrying particles like gluons, photons, and the W and Z bosons. The model accounts for three of the four fundamental forces, excluding gravity. Dr. Shanahan explains that the model has been experimentally verified through the discovery of various particles, with the Higgs boson being the most recent in 2012. The model's equations are concise but require powerful computers to solve, due to their complexity.
🧠 Computational Challenges in Physics
Dr. Shanahan discusses the computational challenges in physics, particularly in calculating properties like the mass of the proton. She mentions the use of supercomputers like Summit to perform these calculations, which would take an infeasible amount of time on standard computers. The lecture also touches on the importance of understanding the standard model to explore physics beyond it, including the study of dark matter and the unification of gravity with the other fundamental forces.
🚀 The Importance of the Proton and Neutron Mass Difference
The small mass difference between the proton and the neutron is discussed, highlighting its importance for the existence of life. Dr. Shanahan explains how recent work has allowed scientists to understand this mass difference in terms of the interplay between strong and electromagnetic interactions. She also talks about the pressure distribution inside a proton, which is a more recent calculation that shows a remarkable level of pressure at the proton's core.
🌟 Nuclear Reactions and the Early Universe
Dr. Shanahan explores the nuclear reactions that occurred in the early universe, such as Big Bang nucleosynthesis, which led to the formation of the first elements. She also discusses how the standard model has been used to study these reactions, providing insights into the underlying quark and gluon dynamics. The lecture touches on the importance of these reactions for understanding the universe's composition and the fundamental processes that govern it.
🌌 Dark Matter and the Structure of the Universe
The existence of dark matter is deduced from various astrophysical observations, such as gravitational lensing and the motion of galaxies. Dr. Shanahan explains that dark matter does not interact with electromagnetic radiation, making it invisible to telescopes. Despite numerous theories, the exact nature of dark matter remains unknown. The lecture also briefly mentions dark energy and its role in the accelerating expansion of the universe.
🔍 Detecting Dark Matter Through Experiments
Three experimental approaches to detecting dark matter are outlined: collider experiments, direct detection, and indirect detection. Dr. Shanahan discusses the challenges in constraining the properties of dark matter particles and the importance of the standard model in these calculations. She also highlights new research on the multi-particle interactions of dark matter, which could have significant implications for interpreting experimental results.
🧮 The Computational Future of Nuclear Physics
Dr. Shanahan emphasizes the need for more intense computations to advance nuclear physics, discussing the limitations imposed by current computational capabilities. She suggests that smarter algorithms, machine learning, and quantum computing may offer ways to overcome these challenges. The lecture also explores the potential of FPGAs to tailor computers for specific calculations, which could significantly speed up the process of understanding complex nuclear physics problems.
🌟 The Standard Model's Success and Future Challenges
The lecture concludes with a reflection on the success of the standard model in describing the universe and the remaining challenges, such as explaining dark matter and the asymmetry between matter and antimatter. Dr. Shanahan expresses hope that the standard model can lead to a deeper understanding of nuclear physics and that it may reveal where it breaks down, pointing towards new physics. The Q&A session addresses questions about the possibility of constructing dark matter from standard model particles, the potential for new forms of matter, and the importance of computational advancements.
👩💼 Career Advice for Aspiring Theoretical Physicists
Dr. Shanahan provides advice for a high school girl interested in theoretical physics, emphasizing the importance of building a strong foundation in mathematics and computer science. She encourages students to understand both pure and applied mathematics and to be comfortable with computing, as these skills are essential for research in fundamental physics.
Mindmap
Keywords
💡Standard Model
💡Quarks
💡Dark Matter
💡Quantum Chromodynamics (QCD)
💡Neutron Star
💡Higgs Boson
💡Proton Radius Puzzle
💡Big Bang Nucleosynthesis
💡Quantum Computing
💡Femtosecond Laser
💡Gravitational Lensing
Highlights
Dr. Fiona Shanahan discusses the fundamental building blocks of the universe, which are quarks and gluons.
The Standard Model of Particle Physics describes 17 fundamental particles and their interactions.
Dr. Shanahan explains the importance of understanding the Standard Model to explore physics beyond it.
Supercomputers are used to perform complex calculations related to the Standard Model that would take millennia to complete on a home computer.
The internal structure of a proton is dynamic and complex, with quark-antiquark pairs emerging from the quantum vacuum.
A small mass difference between the proton and neutron is crucial for the existence of life as we know it.
Recent work has calculated the pressure distribution inside a proton, which is greater than the pressure inside a neutron star at its peak.
The upcoming electron-ion collider in the U.S. is expected to provide higher precision measurements of the internal dynamics of a proton.
The Standard Model describes the primordial nuclear reactions that occurred in the first moments after the Big Bang.
Dr. Shanahan highlights the challenges in unifying the Standard Model with gravity into a single, unified theory.
Dark matter is inferred to exist due to gravitational effects not explained by visible matter, and it is estimated to be five times more abundant than ordinary matter.
The properties of dark matter are still unknown, and various theories propose different mechanisms for its existence.
Experiments to detect dark matter include collider experiments, direct detection, and indirect detection methods.
New calculations suggest that dark matter particles may interact with multiple nucleons at once, which could significantly affect experimental results.
Dr. Shanahan emphasizes the need for more efficient computational methods and algorithms to make progress in understanding the Standard Model and beyond.
Machine learning and artificial intelligence are being explored as potential tools to improve the efficiency of computations in theoretical physics.
Quantum computing and FPGAs (Field-Programmable Gate Arrays) offer new opportunities for tailored computational approaches to complex physics problems.
Dr. Shanahan expresses hope that the Standard Model can explain all of nuclear physics and that it may reveal where it breaks down to point towards new physics.
Transcripts
Browse More Related Video

Neil Turok Public Lecture: The Astonishing Simplicity of Everything
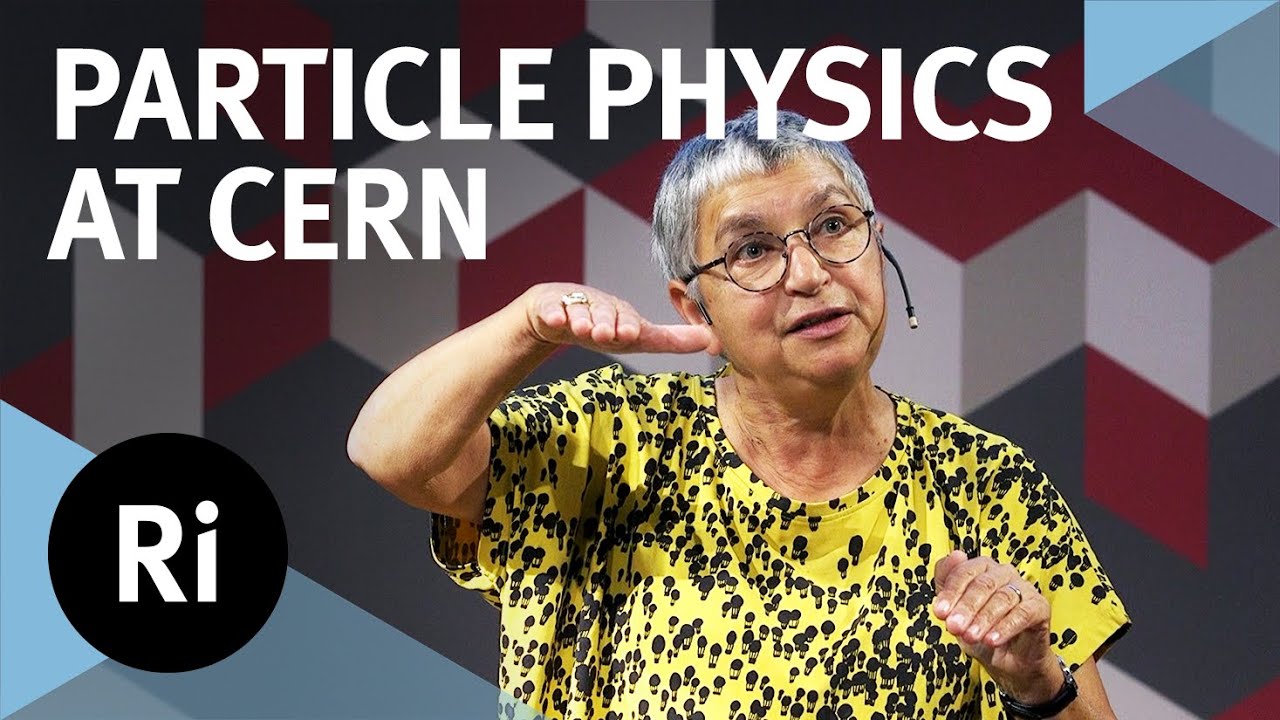
Particle physics made easy - with Pauline Gagnon

Beyond the Higgs: What's Next for the LHC? - with Harry Cliff
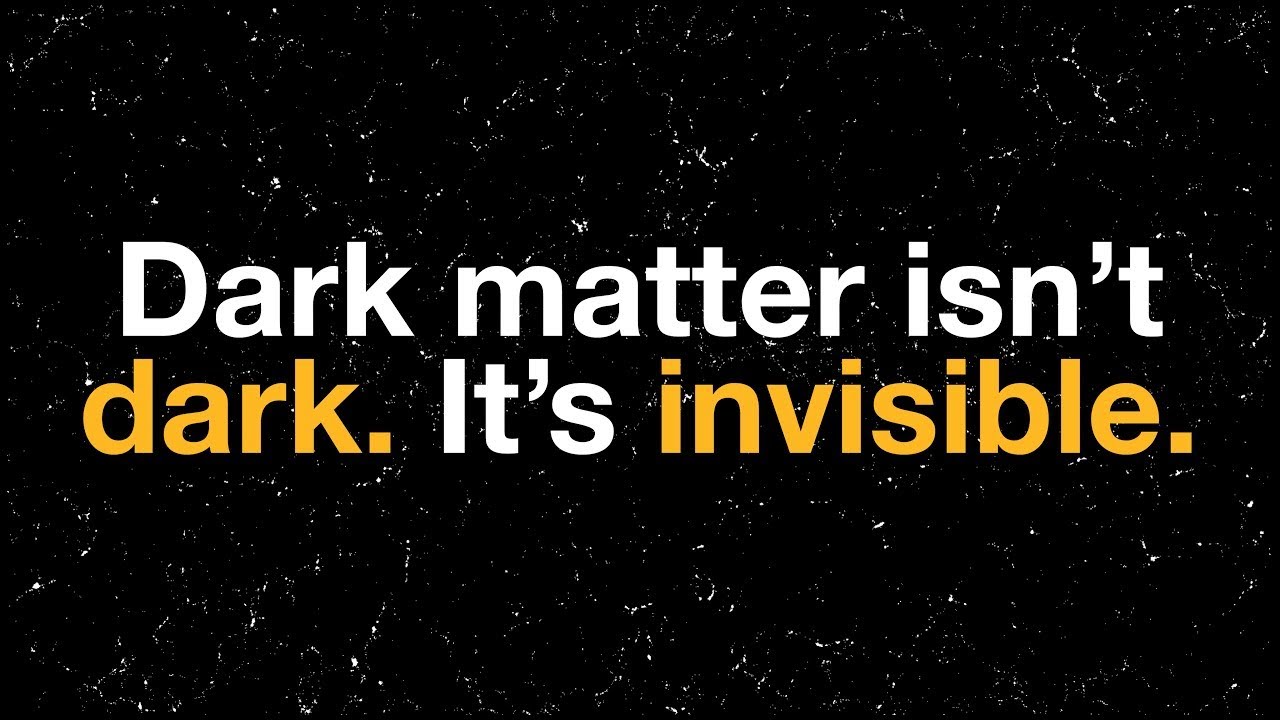
Why we have not discovered dark matter: A theorist’s apology

Muon physics: Have we found a new force of nature? | Alex Keshavarzi | TEDxManchester
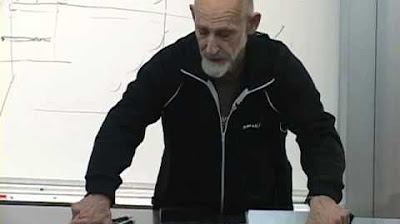
Lecture 1 | Topics in String Theory
5.0 / 5 (0 votes)
Thanks for rating: